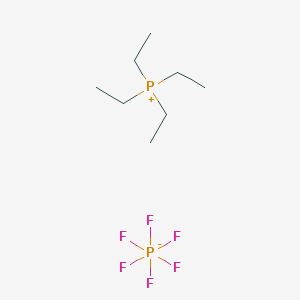
Tetraethylphosphonium hexafluorophosphate
Overview
Description
Tetraethylphosphonium hexafluorophosphate ([P(C₂H₅)₄]PF₆) is a quaternary phosphonium salt composed of a tetraethylphosphonium cation and a hexafluorophosphate (PF₆⁻) anion. This compound is characterized by its high thermal stability, low water solubility, and utility as a non-coordinating ionic species in organic synthesis and catalysis. The PF₆⁻ anion is known for its chemical inertness and weak coordination ability, making it ideal for stabilizing cationic intermediates in polar solvents .
Synthesis typically involves metathesis reactions, where a tetraethylphosphonium halide reacts with a hexafluorophosphate source (e.g., AgPF₆ or NH₄PF₆) to precipitate the desired salt . Applications span catalysis, ionic liquids, and electrochemical systems, leveraging its stability in harsh conditions.
Preparation Methods
Synthetic Routes and Reaction Conditions
Tetraethylphosphonium hexafluorophosphate can be synthesized through the reaction of tetraethylphosphonium chloride with potassium hexafluorophosphate in an organic solvent such as acetonitrile. The reaction is typically carried out under inert gas conditions to prevent moisture from affecting the reaction .
Industrial Production Methods
Industrial production of this compound involves similar synthetic routes but on a larger scale. The process requires careful control of reaction conditions, including temperature, pressure, and the purity of reactants, to ensure high yield and purity of the final product .
Chemical Reactions Analysis
Types of Reactions
Tetraethylphosphonium hexafluorophosphate undergoes various chemical reactions, including:
Oxidation: The compound can be oxidized to form different phosphorus-containing products.
Reduction: It can be reduced under specific conditions to yield phosphine derivatives.
Substitution: The compound can participate in substitution reactions where the hexafluorophosphate anion is replaced by other anions.
Common Reagents and Conditions
Common reagents used in these reactions include oxidizing agents like hydrogen peroxide, reducing agents such as lithium aluminum hydride, and various nucleophiles for substitution reactions. The reactions are typically carried out in organic solvents under controlled temperature and pressure conditions .
Major Products Formed
The major products formed from these reactions depend on the specific reagents and conditions used. For example, oxidation can yield phosphine oxides, while reduction can produce phosphine derivatives .
Scientific Research Applications
Electrolytes in Energy Storage Systems
TEPF is primarily used as an organic electrolyte in high-energy density electric double-layer capacitors (EDLC). Its high conductivity enhances the efficiency of energy storage systems, making it a critical component in modern energy technologies.
Property | Value |
---|---|
Conductivity | High |
Thermal Stability | Stable up to 65 °C |
Solubility | Soluble in polar solvents |
Organic Synthesis
In organic chemistry, TEPF serves as a reagent in various synthetic reactions. It has been utilized for the synthesis of complex organic molecules, including those involved in pharmaceutical development.
Biological Studies
TEPF's interaction with biological membranes has led to its investigation in biochemical studies. It can affect protein solubility through electrostatic interactions, which may have implications for drug delivery systems.
Case Study 1: Antifungal Activity Enhancement
Research demonstrated that phosphonium-modified chitosan derivatives exhibited enhanced antifungal properties compared to unmodified chitosan. Specifically, a derivative with triphenylphosphine showed an inhibition rate of approximately 80% against Phomopsis asparagi at a concentration of 0.5 mg/mL.
Case Study 2: Protein Interaction Studies
A study focused on TEPF's effects on protein solubility revealed its ability to precipitate proteins through electrostatic interactions. This property suggests potential applications in biochemical assays and purification processes.
Case Study 3: Electrolytic Applications
The role of TEPF as an electrolyte in EDLCs was explored, highlighting its capacity to enhance conductivity and stability under various conditions. This characteristic is beneficial for energy storage technologies, particularly in developing efficient capacitors.
Mechanism of Action
The mechanism by which tetraethylphosphonium hexafluorophosphate exerts its effects involves its interaction with molecular targets such as enzymes and cellular membranes. The compound can disrupt membrane integrity and enzyme activity, leading to various biochemical effects. The hexafluorophosphate anion plays a crucial role in these interactions by stabilizing the phosphonium cation and facilitating its entry into cells .
Comparison with Similar Compounds
Comparison with Similar Compounds
Cationic Structural Variations
Hexafluorophosphate salts vary significantly based on cation type, influencing their physical and chemical behaviors:
Key Observations :
- Phosphonium vs. Ammonium : Phosphonium salts exhibit higher thermal stability and lower water solubility compared to ammonium analogs. For example, tetraethylammonium hexafluorophosphate is slightly soluble in water, while phosphonium salts like BOP are more suited for organic-phase reactions .
- Phosphonium vs. Imidazolium : Imidazolium ionic liquids (e.g., 1-butyl-3-methylimidazolium PF₆⁻) are widely used in catalysis but decompose at lower temperatures (~300°C) compared to phosphonium salts, which can withstand >400°C .
Solubility and Conductivity
Hexafluorophosphate salts are generally hydrophobic, but solubility varies with cation size and polarity:
Key Observations :
- Larger cations (e.g., tetrabutylphosphonium) reduce water solubility further due to increased hydrophobicity .
- Imidazolium salts exhibit higher ionic conductivity, making them preferable for electrochemical applications .
Thermal and Chemical Stability
Thermal decomposition temperatures and resistance to hydrolysis are critical for high-temperature processes:
Key Observations :
- Phosphonium salts outperform imidazolium and ammonium salts in thermal stability, ideal for high-temperature catalysis .
- PF₆⁻ salts are generally resistant to hydrolysis but degrade in strongly basic conditions .
Key Observations :
Biological Activity
Tetraethylphosphonium hexafluorophosphate (TEPF) is an organophosphorus compound recognized for its unique chemical properties and potential biological applications. This article provides a comprehensive overview of the biological activity of TEPF, including its mechanisms of action, biochemical pathways, and relevant case studies.
TEPF has the molecular formula C₈H₂₀F₆P₂ and a molecular weight of 292.18 g/mol. It consists of a tetraethylphosphonium cation and a hexafluorophosphate anion, which contributes to its ionic nature and high solubility in polar solvents. The compound appears as a white crystalline solid and is noted for its balance between hydrophobicity from the ethyl groups and hydrophilicity from the hexafluorophosphate anion, making it versatile in various applications.
Interaction with Biological Systems
TEPF is primarily studied for its interactions with biological membranes and proteins. The proposed mechanism involves electrostatic interactions between the positively charged tetraethylphosphonium cation and negatively charged functional groups on protein surfaces. This interaction can lead to protein precipitation, which disrupts their solubility in solution.
Biochemical Pathways
TEPF acts as an organic electrolyte in high-energy density electric double-layer capacitors (EDLC). Its high conductivity is essential for efficient energy production, influencing electron transport chains within biological systems. This property suggests potential applications in drug delivery systems, where TEPF could facilitate the transport of therapeutic compounds across cellular membranes.
Antifungal Properties
Research indicates that phosphonium salts, including TEPF, can enhance antifungal activity when used to modify biopolymers like chitosan. In studies involving chitosan derivatives modified with quaternary phosphonium salts, significant improvements in antifungal efficiency were observed against various plant pathogens, such as Colletotrichum lagenarium and Fusarium oxysporum. For instance, chitosan derivatives exhibited inhibitory indices exceeding 75% at concentrations around 1.0 mg/mL .
Toxicity and Safety Profile
TEPF has been classified as an irritant, particularly to the respiratory tract and eyes. However, it is not considered harmful when ingested based on current evidence. Long-term exposure does not appear to produce chronic adverse health effects, but good hygiene practices are recommended to minimize exposure .
Comparative Analysis with Related Compounds
The following table compares TEPF with other phosphonium salts regarding their molecular structure and biological activity:
Compound Name | Molecular Formula | Unique Features | Biological Activity |
---|---|---|---|
This compound (TEPF) | C₈H₂₀F₆P₂ | Balanced hydrophobicity/hydrophilicity | Protein precipitation, antifungal potential |
Tetrabutylphosphonium hexafluorophosphate | C₁₆H₃₆F₆P₂ | Larger butyl groups affecting solubility | Enhanced solubility in organic reactions |
Triphenylphosphonium hexafluorophosphate | C₁₈H₁₅F₆P | Aromatic character influencing reactivity | Notable antimicrobial properties |
Case Studies
- Antifungal Activity Enhancement : A study demonstrated that quaternary phosphonium-modified chitosan exhibited superior antifungal properties compared to unmodified chitosan. The derivative with triphenylphosphine showed an inhibition rate of approximately 80% against Phomopsis asparagi at 0.5 mg/mL .
- Protein Interaction Studies : Research into TEPF's effects on protein solubility revealed that it could effectively precipitate proteins through electrostatic interactions, suggesting potential applications in biochemical assays.
- Electrolytic Applications : TEPF's role as an electrolyte in EDLCs has been explored, highlighting its ability to enhance conductivity and stability under various conditions, which could be beneficial for energy storage technologies.
Q & A
Basic Research Questions
Q. What are the optimal synthetic methods for preparing Tetraethylphosphonium hexafluorophosphate with high purity?
- The synthesis involves reacting phosphorus pentachloride (PCl₅) with tetraethylphosphonium halides in anhydrous hydrogen fluoride (HF) to form the hexafluorophosphate anion . Alternatively, metathesis reactions using silver hexafluorophosphate (AgPF₆) can precipitate insoluble silver halides, leaving the desired product in solution. Ensure rigorous drying of reagents and conduct reactions under inert atmospheres to minimize hydrolysis. Post-synthesis purification via recrystallization from polar organic solvents (e.g., acetonitrile) enhances purity .
Q. How does the stability of this compound vary under different pH conditions?
- The compound is stable in alkaline conditions but undergoes slow acid-catalyzed hydrolysis to release phosphate ions and HF. For long-term storage, maintain neutral to slightly basic conditions (pH 7–9) and avoid exposure to strong acids. Hydrolysis kinetics can be monitored via conductivity measurements or ³¹P NMR spectroscopy to detect degradation products .
Q. What analytical techniques are recommended for quantifying this compound in solution?
- Gravimetric analysis using tetraphenylarsonium chloride [(C₆H₅)₄As]Cl precipitates the hexafluorophosphate anion for quantification . Spectrophotometric methods with potassium ferricyanide (K₃[Fe(CN)₆]) are also effective, but ensure calibration against standards to account for potential interference from organic cations. Ion chromatography (IC) or ion-selective electrodes may provide complementary data .
Advanced Research Questions
Q. How can this compound be utilized as a non-coordinating electrolyte in electrochemical studies?
- Its low coordinating tendency and high solubility in polar organic solvents (e.g., dichloromethane, propylene carbonate) make it suitable for cyclic voltammetry (CV) and impedance spectroscopy. Use concentrations of 0.1–0.5 M in three-electrode cells with Pt or glassy carbon working electrodes. Pre-purify solvents to eliminate trace water, which can hydrolyze the anion and distort redox potentials .
Q. What experimental design considerations are critical for using this compound in ionic liquid-based catalysis?
- Design biphasic systems to facilitate catalyst recovery, leveraging the compound’s immiscibility with non-polar solvents. Optimize reaction temperatures (<100°C) to maintain ionic liquid stability. Monitor catalyst leaching via inductively coupled plasma mass spectrometry (ICP-MS) and assess recyclability over multiple cycles .
Q. How should researchers mitigate decomposition risks during high-temperature handling of this compound?
- Avoid temperatures >150°C, as thermal degradation releases HF and phosphorus oxyfluorides. Conduct experiments in sealed systems with inert gas purging (e.g., N₂ or Ar). Post-reaction, neutralize residual HF with calcium carbonate (CaCO₃) traps. Use FTIR or Raman spectroscopy to detect decomposition products in real time .
Q. How can conflicting solubility data for this compound in organic solvents be resolved?
- Perform systematic solubility studies using gravimetric analysis under controlled humidity and temperature. Compare results across solvent classes (e.g., acetone vs. THF) and correlate with solvent polarity indices. Conflicting data may arise from residual moisture or impurities; use Karl Fischer titration to verify solvent dryness .
Q. What mechanisms govern the hydrolysis of this compound, and how can they be inhibited?
- Acidic conditions protonate the PF₆⁻ anion, initiating stepwise fluoride loss to form PO₄³⁻. To suppress hydrolysis, buffer solutions to pH >7 and avoid protic solvents. Additives like triethylamine can scavenge free H⁺ ions. Monitor reaction progress via ¹⁹F NMR to track fluoride release .
Q. How does this compound compare to other phosphonium-based ionic liquids in thermal stability?
- Unlike imidazolium-based ionic liquids (e.g., 1,3-dimethylimidazolium PF₆⁻), phosphonium salts exhibit higher thermal stability (decomposition >300°C). Characterize using thermogravimetric analysis (TGA) under N₂ and compare activation energies via Kissinger plots. Note that alkyl chain length impacts stability—bulkier cations (e.g., tetrabutyl) enhance stability .
Q. What spectroscopic methods are most effective for in situ monitoring of this compound degradation?
- Time-resolved FTIR with attenuated total reflection (ATR) accessories can track PF₆⁻ degradation (e.g., loss of ~820 cm⁻¹ peaks). For quantitative analysis, pair with ³¹P NMR to detect phosphate byproducts. Conduct experiments in gloveboxes to prevent atmospheric interference, especially for moisture-sensitive samples .
Properties
IUPAC Name |
tetraethylphosphanium;hexafluorophosphate | |
---|---|---|
Source | PubChem | |
URL | https://pubchem.ncbi.nlm.nih.gov | |
Description | Data deposited in or computed by PubChem | |
InChI |
InChI=1S/C8H20P.F6P/c1-5-9(6-2,7-3)8-4;1-7(2,3,4,5)6/h5-8H2,1-4H3;/q+1;-1 | |
Source | PubChem | |
URL | https://pubchem.ncbi.nlm.nih.gov | |
Description | Data deposited in or computed by PubChem | |
InChI Key |
WJZBUQKNTCKXAE-UHFFFAOYSA-N | |
Source | PubChem | |
URL | https://pubchem.ncbi.nlm.nih.gov | |
Description | Data deposited in or computed by PubChem | |
Canonical SMILES |
CC[P+](CC)(CC)CC.F[P-](F)(F)(F)(F)F | |
Source | PubChem | |
URL | https://pubchem.ncbi.nlm.nih.gov | |
Description | Data deposited in or computed by PubChem | |
Molecular Formula |
C8H20F6P2 | |
Source | PubChem | |
URL | https://pubchem.ncbi.nlm.nih.gov | |
Description | Data deposited in or computed by PubChem | |
DSSTOX Substance ID |
DTXSID60375226 | |
Record name | Tetraethylphosphonium hexafluorophosphate | |
Source | EPA DSSTox | |
URL | https://comptox.epa.gov/dashboard/DTXSID60375226 | |
Description | DSSTox provides a high quality public chemistry resource for supporting improved predictive toxicology. | |
Molecular Weight |
292.18 g/mol | |
Source | PubChem | |
URL | https://pubchem.ncbi.nlm.nih.gov | |
Description | Data deposited in or computed by PubChem | |
CAS No. |
111928-07-5 | |
Record name | Tetraethylphosphonium hexafluorophosphate | |
Source | EPA DSSTox | |
URL | https://comptox.epa.gov/dashboard/DTXSID60375226 | |
Description | DSSTox provides a high quality public chemistry resource for supporting improved predictive toxicology. | |
Record name | Tetraethylphosphonium Hexafluorophosphate | |
Source | European Chemicals Agency (ECHA) | |
URL | https://echa.europa.eu/information-on-chemicals | |
Description | The European Chemicals Agency (ECHA) is an agency of the European Union which is the driving force among regulatory authorities in implementing the EU's groundbreaking chemicals legislation for the benefit of human health and the environment as well as for innovation and competitiveness. | |
Explanation | Use of the information, documents and data from the ECHA website is subject to the terms and conditions of this Legal Notice, and subject to other binding limitations provided for under applicable law, the information, documents and data made available on the ECHA website may be reproduced, distributed and/or used, totally or in part, for non-commercial purposes provided that ECHA is acknowledged as the source: "Source: European Chemicals Agency, http://echa.europa.eu/". Such acknowledgement must be included in each copy of the material. ECHA permits and encourages organisations and individuals to create links to the ECHA website under the following cumulative conditions: Links can only be made to webpages that provide a link to the Legal Notice page. | |
Retrosynthesis Analysis
AI-Powered Synthesis Planning: Our tool employs the Template_relevance Pistachio, Template_relevance Bkms_metabolic, Template_relevance Pistachio_ringbreaker, Template_relevance Reaxys, Template_relevance Reaxys_biocatalysis model, leveraging a vast database of chemical reactions to predict feasible synthetic routes.
One-Step Synthesis Focus: Specifically designed for one-step synthesis, it provides concise and direct routes for your target compounds, streamlining the synthesis process.
Accurate Predictions: Utilizing the extensive PISTACHIO, BKMS_METABOLIC, PISTACHIO_RINGBREAKER, REAXYS, REAXYS_BIOCATALYSIS database, our tool offers high-accuracy predictions, reflecting the latest in chemical research and data.
Strategy Settings
Precursor scoring | Relevance Heuristic |
---|---|
Min. plausibility | 0.01 |
Model | Template_relevance |
Template Set | Pistachio/Bkms_metabolic/Pistachio_ringbreaker/Reaxys/Reaxys_biocatalysis |
Top-N result to add to graph | 6 |
Feasible Synthetic Routes
Disclaimer and Information on In-Vitro Research Products
Please be aware that all articles and product information presented on BenchChem are intended solely for informational purposes. The products available for purchase on BenchChem are specifically designed for in-vitro studies, which are conducted outside of living organisms. In-vitro studies, derived from the Latin term "in glass," involve experiments performed in controlled laboratory settings using cells or tissues. It is important to note that these products are not categorized as medicines or drugs, and they have not received approval from the FDA for the prevention, treatment, or cure of any medical condition, ailment, or disease. We must emphasize that any form of bodily introduction of these products into humans or animals is strictly prohibited by law. It is essential to adhere to these guidelines to ensure compliance with legal and ethical standards in research and experimentation.