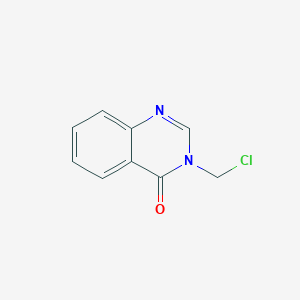
3-(chloromethyl)quinazolin-4(3H)-one
Overview
Description
3-(chloromethyl)quinazolin-4(3H)-one is a derivative of quinazolinone, a heterocyclic compound that has significant importance in medicinal chemistry . Quinazolinones possess a wide spectrum of biological properties, including antibacterial, antifungal, anticonvulsant, anti-inflammatory, anti-HIV, anticancer, and analgesic activities .
Synthesis Analysis
Quinazolin-4(3H)-ones have been synthesized through various methods. One approach involves the one-pot condensation of anthranilic acid, trimethyl orthoformate, and primary amines . Another method involves the use of a two-module nonribosomal peptide synthase (NRPS) that catalyzes tripeptide formation .Molecular Structure Analysis
Quinazolinones can be classified into five categories based on the substitution patterns of the ring system . These include 2-substituted-4(3H)-quinazolinones, 3-substituted-4(3H)-quinazolinones, 4-substituted-quinazolines, 2,3-disubstituted-4(3H)-quinazolinones, and 2,4-disubstituted-4(3H)-quinazolinones .Chemical Reactions Analysis
Quinazolinone derivatives have shown to possess antibacterial activities, especially against gram-positive strains, and fungi through their interaction with the cell wall and DNA structures . Substitution at positions 2 and 3, existence of a halogen atom at positions 6 and 8, and substitution (mainly amine or substituted amine) at the 4th position of the quinazolinone ring can improve their antimicrobial activities .Physical and Chemical Properties Analysis
The physical and chemical properties of this compound derivatives can vary depending on the specific substitutions made on the quinazolinone ring . For instance, 3-(5-chloro-2-methylphenyl)-2-(chloromethyl)quinazolin-4(3H)-one is a white powder with a melting point of 157 °C .Scientific Research Applications
Antioxidant and Cytotoxic Activity : Derivatives of quinazolin-4(3H)-one, including those with polyphenolic compounds, exhibit significant antioxidant and cytotoxic activities. They demonstrate high cytotoxicity against cancerous cell lines and compatibility with normal cells, suggesting potential in cancer treatment (Pele et al., 2022).
Antimicrobial Activity : Certain polyhalobenzonitrile quinazolin-4(3H)-one derivatives have shown substantial antimicrobial activities against various bacteria and fungi strains, indicating their potential as antimicrobial agents (Shi et al., 2013).
Quantitative Determination Methods : Research has been conducted to develop UV-spectrophotometry methods for the quantitative determination of quinazolin-4(3H)-on derivatives, aiding in the quality control of these substances (Mishchenko et al., 2020).
Anticonvulsant Activity : Studies have shown that certain 3-substituted-2-(substituted-phenoxymethyl) quinazolin-4(3H)-one derivatives exhibit moderate to significant anticonvulsant activities, suggesting their potential use in treating seizure disorders (Georgey et al., 2008).
Antiviral Activities : Novel 3-sulphonamido-quinazolin-4(3H)-One derivatives have been synthesized and evaluated for antiviral activities against various respiratory and biodefense viruses, including influenza and dengue, indicating their potential in antiviral therapy (Selvam et al., 2007).
Anti-Tuberculosis Activity : Certain 2,3-disubstituted quinazolin-4(1H)-ones have exhibited potent anti-tubercular activities against Mycobacterium tuberculosis, indicating their potential application in treating multi-drug resistant tuberculosis (Kumar et al., 2021).
Analgesic and Anti-inflammatory Activities : Some derivatives of quinazolin-4(3H)-one, like 3-ethyl-2-substituted amino-quinazolin-4(3H)-ones, have shown significant analgesic and anti-inflammatory activities, suggesting their potential in pain and inflammation management (Sheorey et al., 2013).
Antitumor Activity : Several studies have demonstrated the antitumor potential of quinazolin-4(3H)-one derivatives against various cancer cell lines, including leukemia and mammary tumor cells (Perchellet et al., 2011).
Mechanism of Action
Target of Action
Quinazolinone derivatives are known to have broad applications, including antimalarial, antitumor, anticonvulsant, fungicidal, antimicrobial, and anti-inflammatory effects . These effects suggest that the compound may interact with a variety of biological targets.
Mode of Action
Given the broad range of biological activities associated with quinazolinone derivatives, it is likely that the compound interacts with its targets in a way that modulates their function, leading to the observed effects .
Biochemical Pathways
The broad range of biological activities associated with quinazolinone derivatives suggests that the compound may impact multiple pathways, leading to downstream effects such as antimalarial, antitumor, anticonvulsant, fungicidal, antimicrobial, and anti-inflammatory activities .
Result of Action
The compound’s broad range of biological activities suggests that it may induce a variety of effects at the molecular and cellular levels .
Future Directions
The future directions in the research of 3-(chloromethyl)quinazolin-4(3H)-one could involve the design and synthesis of new derivatives with improved biological activities. Given the wide range of biological properties exhibited by quinazolinone derivatives, there is significant potential for the development of new therapeutic agents .
Biochemical Analysis
Biochemical Properties
3-(chloromethyl)quinazolin-4(3H)-one plays a crucial role in several biochemical reactions. It interacts with a variety of enzymes, proteins, and other biomolecules, influencing their activity and function. For instance, this compound has been shown to inhibit certain kinases, which are enzymes that transfer phosphate groups from high-energy donor molecules to specific substrates . This inhibition can affect various signaling pathways within the cell, leading to altered cellular responses.
Additionally, this compound can bind to DNA and RNA, potentially interfering with the replication and transcription processes . This binding is often facilitated by the compound’s ability to form hydrogen bonds and hydrophobic interactions with nucleic acids. The interactions between this compound and these biomolecules are critical for understanding its biochemical properties and potential therapeutic applications.
Cellular Effects
The effects of this compound on various cell types and cellular processes are profound. This compound can influence cell function by modulating cell signaling pathways, gene expression, and cellular metabolism. For example, this compound has been observed to induce apoptosis in cancer cells by activating pro-apoptotic pathways and inhibiting anti-apoptotic signals . This selective induction of cell death in cancer cells highlights its potential as an anticancer agent.
Moreover, this compound can affect gene expression by binding to transcription factors and altering their activity . This can lead to changes in the expression levels of various genes, impacting cellular processes such as proliferation, differentiation, and metabolism. The compound’s influence on cellular metabolism is also noteworthy, as it can inhibit key metabolic enzymes, leading to reduced energy production and altered metabolic flux .
Molecular Mechanism
The molecular mechanism of action of this compound involves several key interactions at the molecular level. One of the primary mechanisms is the inhibition of enzyme activity through direct binding to the enzyme’s active site . This binding can prevent the enzyme from interacting with its natural substrate, thereby inhibiting its catalytic activity. For example, this compound has been shown to inhibit tyrosine kinases, which play a critical role in cell signaling and growth .
In addition to enzyme inhibition, this compound can also modulate gene expression by interacting with transcription factors and other regulatory proteins . This interaction can lead to changes in the transcriptional activity of specific genes, resulting in altered cellular responses. Furthermore, the compound’s ability to bind to nucleic acids can interfere with DNA replication and RNA transcription, further contributing to its molecular mechanism of action .
Temporal Effects in Laboratory Settings
The temporal effects of this compound in laboratory settings are influenced by its stability, degradation, and long-term effects on cellular function. Studies have shown that this compound is relatively stable under standard laboratory conditions, with minimal degradation over time . Its stability can be affected by factors such as pH, temperature, and exposure to light.
Over extended periods, this compound can exert long-term effects on cellular function, including sustained inhibition of enzyme activity and prolonged changes in gene expression . These long-term effects are particularly relevant in in vitro and in vivo studies, where the compound’s impact on cellular processes can be observed over time.
Dosage Effects in Animal Models
The effects of this compound vary with different dosages in animal models. At lower doses, the compound may exhibit minimal toxicity and primarily exert its therapeutic effects, such as inhibiting tumor growth or reducing inflammation . At higher doses, this compound can cause toxic or adverse effects, including hepatotoxicity, nephrotoxicity, and gastrointestinal disturbances .
Threshold effects have also been observed, where a specific dosage is required to achieve a therapeutic response. Beyond this threshold, increasing the dosage may not significantly enhance the therapeutic effect but can increase the risk of adverse effects . Understanding the dosage effects of this compound is crucial for optimizing its use in therapeutic applications.
Metabolic Pathways
This compound is involved in several metabolic pathways, interacting with various enzymes and cofactors. One of the primary metabolic pathways involves the cytochrome P450 enzyme system, which is responsible for the oxidative metabolism of many xenobiotics . This system can metabolize this compound into various metabolites, some of which may retain biological activity.
Additionally, this compound can affect metabolic flux by inhibiting key metabolic enzymes, such as those involved in glycolysis and the tricarboxylic acid cycle . This inhibition can lead to altered levels of metabolites and reduced energy production, impacting cellular metabolism and function.
Transport and Distribution
The transport and distribution of this compound within cells and tissues are mediated by various transporters and binding proteins. For example, the compound can be transported across cell membranes by specific transporters, such as ATP-binding cassette (ABC) transporters . Once inside the cell, this compound can bind to intracellular proteins, influencing its localization and accumulation.
The distribution of this compound within tissues is also influenced by factors such as blood flow, tissue permeability, and binding to plasma proteins . These factors can affect the compound’s bioavailability and therapeutic efficacy, highlighting the importance of understanding its transport and distribution properties.
Subcellular Localization
The subcellular localization of this compound is critical for its activity and function. This compound can be directed to specific cellular compartments or organelles through targeting signals or post-translational modifications . For instance, this compound may be localized to the nucleus, where it can interact with DNA and transcription factors, influencing gene expression.
Additionally, the compound can be localized to the mitochondria, where it can affect mitochondrial function and energy production . The subcellular localization of this compound is essential for understanding its mechanism of action and potential therapeutic applications.
Properties
IUPAC Name |
3-(chloromethyl)quinazolin-4-one | |
---|---|---|
Source | PubChem | |
URL | https://pubchem.ncbi.nlm.nih.gov | |
Description | Data deposited in or computed by PubChem | |
InChI |
InChI=1S/C9H7ClN2O/c10-5-12-6-11-8-4-2-1-3-7(8)9(12)13/h1-4,6H,5H2 | |
Source | PubChem | |
URL | https://pubchem.ncbi.nlm.nih.gov | |
Description | Data deposited in or computed by PubChem | |
InChI Key |
RXIWZRBBBSBWBG-UHFFFAOYSA-N | |
Source | PubChem | |
URL | https://pubchem.ncbi.nlm.nih.gov | |
Description | Data deposited in or computed by PubChem | |
Canonical SMILES |
C1=CC=C2C(=C1)C(=O)N(C=N2)CCl | |
Source | PubChem | |
URL | https://pubchem.ncbi.nlm.nih.gov | |
Description | Data deposited in or computed by PubChem | |
Molecular Formula |
C9H7ClN2O | |
Source | PubChem | |
URL | https://pubchem.ncbi.nlm.nih.gov | |
Description | Data deposited in or computed by PubChem | |
Molecular Weight |
194.62 g/mol | |
Source | PubChem | |
URL | https://pubchem.ncbi.nlm.nih.gov | |
Description | Data deposited in or computed by PubChem | |
Retrosynthesis Analysis
AI-Powered Synthesis Planning: Our tool employs the Template_relevance Pistachio, Template_relevance Bkms_metabolic, Template_relevance Pistachio_ringbreaker, Template_relevance Reaxys, Template_relevance Reaxys_biocatalysis model, leveraging a vast database of chemical reactions to predict feasible synthetic routes.
One-Step Synthesis Focus: Specifically designed for one-step synthesis, it provides concise and direct routes for your target compounds, streamlining the synthesis process.
Accurate Predictions: Utilizing the extensive PISTACHIO, BKMS_METABOLIC, PISTACHIO_RINGBREAKER, REAXYS, REAXYS_BIOCATALYSIS database, our tool offers high-accuracy predictions, reflecting the latest in chemical research and data.
Strategy Settings
Precursor scoring | Relevance Heuristic |
---|---|
Min. plausibility | 0.01 |
Model | Template_relevance |
Template Set | Pistachio/Bkms_metabolic/Pistachio_ringbreaker/Reaxys/Reaxys_biocatalysis |
Top-N result to add to graph | 6 |
Feasible Synthetic Routes
Disclaimer and Information on In-Vitro Research Products
Please be aware that all articles and product information presented on BenchChem are intended solely for informational purposes. The products available for purchase on BenchChem are specifically designed for in-vitro studies, which are conducted outside of living organisms. In-vitro studies, derived from the Latin term "in glass," involve experiments performed in controlled laboratory settings using cells or tissues. It is important to note that these products are not categorized as medicines or drugs, and they have not received approval from the FDA for the prevention, treatment, or cure of any medical condition, ailment, or disease. We must emphasize that any form of bodily introduction of these products into humans or animals is strictly prohibited by law. It is essential to adhere to these guidelines to ensure compliance with legal and ethical standards in research and experimentation.