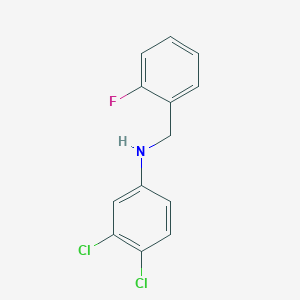
3,4-dichloro-N-(2-fluorobenzyl)aniline
- Click on QUICK INQUIRY to receive a quote from our team of experts.
- With the quality product at a COMPETITIVE price, you can focus more on your research.
Overview
Description
3,4-Dichloro-N-(2-fluorobenzyl)aniline: is an organic compound with the molecular formula C13H10Cl2FN . It consists of an aniline ring substituted with two chlorine atoms at the 3 and 4 positions and a fluorobenzyl group at the nitrogen atom.
Preparation Methods
Synthetic Routes and Reaction Conditions: The synthesis of 3,4-dichloro-N-(2-fluorobenzyl)aniline typically involves the reaction of 3,4-dichloroaniline with 2-fluorobenzyl chloride under basic conditions. The reaction is usually carried out in the presence of a base such as sodium hydroxide or potassium carbonate, which facilitates the nucleophilic substitution of the chlorine atom by the aniline nitrogen .
Industrial Production Methods: Industrial production methods for this compound are not well-documented in the public domain. the general approach would involve scaling up the laboratory synthesis methods, optimizing reaction conditions, and ensuring the purity and yield of the final product through various purification techniques such as recrystallization or chromatography.
Chemical Reactions Analysis
Types of Reactions: 3,4-Dichloro-N-(2-fluorobenzyl)aniline can undergo several types of chemical reactions, including:
Nucleophilic Substitution: The chlorine atoms on the aniline ring can be substituted by various nucleophiles.
Oxidation and Reduction: The compound can undergo oxidation and reduction reactions, altering the oxidation state of the nitrogen atom.
Coupling Reactions: It can participate in coupling reactions such as Suzuki-Miyaura coupling, forming new carbon-carbon bonds.
Common Reagents and Conditions:
Nucleophilic Substitution: Reagents such as sodium hydroxide or potassium carbonate in a suitable solvent.
Oxidation: Oxidizing agents like potassium permanganate or hydrogen peroxide.
Reduction: Reducing agents such as lithium aluminum hydride or sodium borohydride.
Coupling Reactions: Palladium catalysts and organoboron reagents under mild conditions.
Major Products: The major products formed from these reactions depend on the specific reagents and conditions used. For example, nucleophilic substitution can yield various substituted anilines, while coupling reactions can produce biaryl compounds.
Scientific Research Applications
Chemistry: In chemistry, 3,4-dichloro-N-(2-fluorobenzyl)aniline is used as an intermediate in the synthesis of more complex organic molecules. It is particularly useful in the development of pharmaceuticals and agrochemicals .
Biology: The compound may be used in biological research to study the effects of halogenated anilines on biological systems. Its structural features make it a candidate for investigating interactions with enzymes and receptors.
Industry: In industry, this compound can be used in the production of dyes, pigments, and other specialty chemicals .
Mechanism of Action
The mechanism of action of 3,4-dichloro-N-(2-fluorobenzyl)aniline is not explicitly detailed in the literature. based on its structure, it is likely to interact with biological targets through hydrogen bonding, hydrophobic interactions, and halogen bonding. These interactions can influence the activity of enzymes, receptors, and other proteins, leading to various biological effects.
Comparison with Similar Compounds
- 2,3-Dichloroaniline
- 2,4-Dichloroaniline
- 2,5-Dichloroaniline
- 2,6-Dichloroaniline
- 3,4-Dichloroaniline
- 3,5-Dichloroaniline
Comparison: 3,4-Dichloro-N-(2-fluorobenzyl)aniline is unique due to the presence of both chlorine and fluorine substituents, which can significantly influence its chemical reactivity and biological activity. The fluorobenzyl group adds a level of complexity and potential for specific interactions that are not present in simpler dichloroanilines.
Biological Activity
3,4-Dichloro-N-(2-fluorobenzyl)aniline is an organic compound with the molecular formula C₁₃H₁₀Cl₂FN. Its structure features a dichloro-substituted aniline core with a fluorobenzyl group attached to the nitrogen atom. This compound has garnered attention for its potential applications in pharmaceuticals and agrochemicals, attributed to its unique chemical properties and biological activities.
Antimicrobial Properties
Recent studies have indicated that compounds structurally similar to this compound exhibit significant antimicrobial activity. For instance, derivatives with similar aniline structures have been evaluated for their effectiveness against various pathogens. In one study, compounds demonstrated promising antibacterial activity against strains such as E. coli, P. aeruginosa, B. subtilis, and S. aureus, with some showing better efficacy than standard antibiotics like ciprofloxacin .
The biological activity of this compound can be attributed to its ability to interact with specific biological targets:
- Protein Binding : Interaction studies have shown that this compound binds effectively to certain proteins and enzymes, influencing their activity and potentially leading to therapeutic effects.
- Biofilm Inhibition : Similar compounds have been reported to inhibit biofilm formation in pathogenic fungi such as Candida albicans, indicating that this compound may also possess antifungal properties .
Structure-Activity Relationship (SAR)
Understanding the structure-activity relationship is crucial for optimizing the biological activity of this compound. The arrangement of chlorine and fluorine atoms on the benzene ring significantly impacts its reactivity and biological interactions. For example, variations in substituent positions can alter binding affinities and biological effects.
Compound Name | Molecular Formula | Unique Features |
---|---|---|
3,4-Dichloro-N-(4-fluorobenzyl)aniline | C₁₃H₁₀Cl₂FN | Different position of fluorine substituent |
2,4-Dichloro-N-(4-fluorobenzyl)aniline | C₁₃H₁₀Cl₂FN | Variation in chlorine positions |
3-Fluoro-N-(2-chlorobenzyl)aniline | C₁₃H₁₀ClF | Contains only one chlorine substituent |
3-Chloro-N-(2-fluorobenzyl)aniline | C₁₃H₁₀ClFN | Different halogen substitutions affecting reactivity |
Case Study 1: Antifungal Activity
In a study evaluating antifungal agents, derivatives of aniline were synthesized and tested for their ability to inhibit biofilm formation by C. albicans. The results showed that certain compounds had IC50 values comparable to established antifungal treatments like fluconazole, suggesting that modifications similar to those in this compound could enhance antifungal efficacy .
Case Study 2: Antibacterial Screening
Another investigation focused on the antibacterial properties of aniline derivatives against multiple bacterial strains. Compounds were screened for minimum inhibitory concentration (MIC), revealing that several exhibited stronger antibacterial effects than traditional antibiotics against B. subtilis. This highlights the potential of compounds like this compound in developing new antimicrobial agents .
Molecular Docking Studies
Molecular docking studies have been employed to understand the binding interactions between this compound and target proteins involved in microbial resistance. These studies provide insights into the mechanisms by which this compound may exert its biological effects and help guide further modifications for improved potency .
Properties
IUPAC Name |
3,4-dichloro-N-[(2-fluorophenyl)methyl]aniline |
Source
|
---|---|---|
Source | PubChem | |
URL | https://pubchem.ncbi.nlm.nih.gov | |
Description | Data deposited in or computed by PubChem | |
InChI |
InChI=1S/C13H10Cl2FN/c14-11-6-5-10(7-12(11)15)17-8-9-3-1-2-4-13(9)16/h1-7,17H,8H2 |
Source
|
Source | PubChem | |
URL | https://pubchem.ncbi.nlm.nih.gov | |
Description | Data deposited in or computed by PubChem | |
InChI Key |
ZLTRTUPFZYDHAG-UHFFFAOYSA-N |
Source
|
Source | PubChem | |
URL | https://pubchem.ncbi.nlm.nih.gov | |
Description | Data deposited in or computed by PubChem | |
Canonical SMILES |
C1=CC=C(C(=C1)CNC2=CC(=C(C=C2)Cl)Cl)F |
Source
|
Source | PubChem | |
URL | https://pubchem.ncbi.nlm.nih.gov | |
Description | Data deposited in or computed by PubChem | |
Molecular Formula |
C13H10Cl2FN |
Source
|
Source | PubChem | |
URL | https://pubchem.ncbi.nlm.nih.gov | |
Description | Data deposited in or computed by PubChem | |
Molecular Weight |
270.13 g/mol |
Source
|
Source | PubChem | |
URL | https://pubchem.ncbi.nlm.nih.gov | |
Description | Data deposited in or computed by PubChem | |
Disclaimer and Information on In-Vitro Research Products
Please be aware that all articles and product information presented on BenchChem are intended solely for informational purposes. The products available for purchase on BenchChem are specifically designed for in-vitro studies, which are conducted outside of living organisms. In-vitro studies, derived from the Latin term "in glass," involve experiments performed in controlled laboratory settings using cells or tissues. It is important to note that these products are not categorized as medicines or drugs, and they have not received approval from the FDA for the prevention, treatment, or cure of any medical condition, ailment, or disease. We must emphasize that any form of bodily introduction of these products into humans or animals is strictly prohibited by law. It is essential to adhere to these guidelines to ensure compliance with legal and ethical standards in research and experimentation.