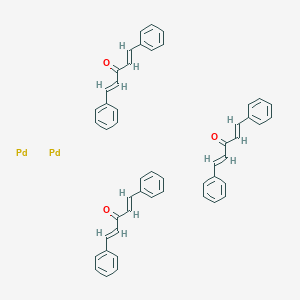
Tris(dibenzylideneacetone)dipalladium(0)
Overview
Description
Tris(dibenzylideneacetone)dipalladium(0) ([Pd₂(dba)₃]) is a homoleptic palladium(0) complex with three dibenzylideneacetone (dba) ligands. It is a dark purple/brown crystalline solid, sparingly soluble in organic solvents, and widely used as a catalyst in cross-coupling reactions (e.g., Suzuki, Heck, Stille) due to its labile dba ligands, which facilitate ligand displacement during catalytic cycles .
Preparation Methods
Tris DBA is typically prepared from dibenzylideneacetone and sodium tetrachloropalladate. The process involves the reaction of these two compounds, followed by recrystallization from chloroform. The complex is often supplied as the adduct [Pd2(dba)3·CHCl3] due to its common recrystallization from chloroform . Industrial production methods may involve scaling up this process, although challenges such as the solubility of intermediates and the need for large amounts of solvents must be addressed .
Chemical Reactions Analysis
Tris DBA undergoes various types of reactions, primarily serving as a source of soluble palladium (0) in catalytic processes. It is particularly effective in facilitating oxidative addition reactions. Common reactions involving Tris DBA include:
- Negishi coupling
- Suzuki coupling
- Carroll rearrangement
- Trost asymmetric allylic alkylation
- Buchwald–Hartwig amination
The reagents and conditions used in these reactions vary, but they typically involve the use of aryl halides and boronic acids in the presence of Tris DBA as a catalyst. The major products formed from these reactions are often complex organic molecules used in pharmaceuticals and materials science .
Scientific Research Applications
Chemical Properties and Structure
Tris(dibenzylideneacetone)dipalladium(0) is a dark purple solid with the chemical formula CHOPd and a molecular weight of approximately 916.12 g/mol. It is typically synthesized from dibenzylideneacetone and sodium tetrachloropalladate. The compound is often supplied as a chloroform adduct, Pd(dba)·CHCl, which enhances its solubility in organic solvents, facilitating its use in homogeneous catalysis .
Catalysis
Cross-Coupling Reactions
Pd(dba) is predominantly employed as a catalyst in cross-coupling reactions, such as:
- Suzuki Coupling : Facilitates the formation of biaryl compounds by coupling aryl halides with boronic acids.
- Heck Reaction : Used for the coupling of alkenes with aryl halides to form substituted alkenes.
- Buchwald-Hartwig Amination : Enables the formation of amines from aryl halides and amines.
These reactions are crucial for synthesizing complex organic molecules in pharmaceuticals and agrochemicals .
Organic Synthesis
The capability of Pd(dba) to form carbon-carbon bonds makes it essential for developing new drugs and agrochemicals. It allows researchers to create diverse molecular architectures, thereby expanding the library of potential therapeutic agents .
Material Science
In material science, Pd(dba) plays a role in producing advanced materials, including:
- Nanomaterials : Enhances properties like conductivity and strength.
- Polymers : Contributes to the development of high-performance polymers used in electronics and structural applications .
Research in Homogeneous Catalysis
This compound serves as a model for studying reaction mechanisms and catalyst design, providing insights that can lead to improved efficiency and selectivity in chemical processes. Such research is critical for advancing sustainable chemistry practices .
Case Study 1: Suzuki Coupling of Aryl Halides
A study demonstrated the efficiency of Pd(dba) in catalyzing Suzuki couplings between various aryl halides and boronic acids, achieving yields above 90% under optimized conditions. The reaction conditions included a base (such as KCO) and an organic solvent (like toluene) .
Case Study 2: Heck Reaction for Alkene Synthesis
In another investigation, Pd(dba) was utilized to couple styrene derivatives with aryl halides, resulting in high yields of substituted alkenes. The reaction was carried out under mild conditions, showcasing the catalyst's versatility and effectiveness .
Case Study 3: Development of Chiral Drugs
Research highlighted the use of Pd(dba) in asymmetric synthesis pathways leading to chiral drugs. The catalyst facilitated key steps that resulted in high enantioselectivity, essential for producing pharmacologically active compounds with desired stereochemistry .
Data Table: Summary of Applications
Application Area | Specific Reactions | Key Benefits |
---|---|---|
Catalysis | Suzuki Coupling | High yield synthesis of biaryl compounds |
Heck Reaction | Formation of substituted alkenes | |
Buchwald-Hartwig Amination | Efficient amine synthesis | |
Organic Synthesis | Carbon-Carbon Bond Formation | Diverse molecular architectures for drug development |
Material Science | Nanomaterial Production | Enhanced conductivity and strength |
Polymer Development | High-performance materials for electronics | |
Research | Mechanistic Studies | Insights into catalyst efficiency and selectivity |
Mechanism of Action
The mechanism of action of Tris DBA involves its ability to inhibit several key signaling pathways. It has been shown to inhibit the activation of mitogen-activated protein kinase (MAPK), Akt, and STAT3, which are involved in cell proliferation and survival. Tris DBA also inhibits N-myristoyltransferase-1, a key enzyme required for the optimal activity of membrane-based signaling molecules . This inhibition leads to reduced cell proliferation and increased apoptosis in cancer cells.
Comparison with Similar Compounds
Table 1: Key Palladium(0) Catalysts and Their Properties
Key Findings :
- Reactivity : [Pd₂(dba)₃] exhibits superior reactivity in coupling reactions compared to [Pd(PPh₃)₄] due to its labile dba ligands, which readily dissociate to form active Pd(0) species .
- Catalyst Preparation : [Pd₂(dba)₃] enables rapid (<5 minutes) synthesis of Pd/C catalysts via direct deposition, outperforming traditional Pd(II) precursors like Pd(OAc)₂ in efficiency .
- Solubility : Unlike [Pd(PPh₃)₄], which is soluble in aromatic solvents, [Pd₂(dba)₃] is sparingly soluble in most solvents, limiting its use in homogeneous catalysis without additional ligands .
Table 2: Therapeutic Profile of Tris DBA vs. Other Compounds
Key Findings :
- STAT3 Inhibition : Tris DBA uniquely upregulates SHP2 phosphatase, promoting STAT3 dephosphorylation, a mechanism distinct from direct STAT3-binding inhibitors .
- Translational Potential: Tris DBA’s efficacy in orthotopic HCC and MM models supports its clinical candidacy, whereas other palladium complexes (e.g., Pd(II) drugs) remain underexplored in oncology .
Physical and Chemical Properties
Table 3: Physicochemical Comparison
Property | Tris(dibenzylideneacetone)dipalladium(0) | Tetrakis(triphenylphosphine)palladium(0) | Bis(dibenzylideneacetone)palladium(0) |
---|---|---|---|
Molecular Formula | Pd₂(C₁₇H₁₄O)₃ | Pd(PPh₃)₄ | Pd(C₁₇H₁₄O)₂ |
Molecular Weight (g/mol) | 915.72 | 1155.56 | 575.00 (approx.) |
Melting Point | 152–155°C | 103–107°C (decomposes) | 120–125°C |
Solubility | Insoluble in water; soluble in THF, DCM | Soluble in benzene, toluene | Similar to [Pd₂(dba)₃] |
Pd Content | ~23.2% | ~9.2% | ~18.5% |
Stability | Air-sensitive | Air-stable under inert conditions | Moderately air-sensitive |
References |
Biological Activity
Tris(dibenzylideneacetone)dipalladium(0), commonly referred to as Pd(dba), is a palladium complex that has garnered attention due to its notable biological activities, particularly in the fields of oncology and enzymatic inhibition. This article explores the compound's biological properties, mechanisms of action, and relevant case studies.
- Molecular Formula : CHOPd
- Molecular Weight : 915.72 g/mol
- Melting Point : 152-155°C
- Solubility : Insoluble in water
Tris(dibenzylideneacetone)dipalladium(0) acts primarily as a catalyst in various chemical reactions, but its biological activity is largely attributed to its role as an inhibitor of specific enzymes. Notably, it has been identified as an inhibitor of N-myristoyltransferase-1 (NMT-1), which is crucial for the post-translational modification of proteins involved in cell signaling and proliferation .
Antitumor Activity
Numerous studies have reported the antitumor effects of Tris(dibenzylideneacetone)dipalladium(0). For instance:
- In Vivo Studies : It demonstrated significant antitumor activity against melanoma in animal models, indicating its potential as a therapeutic agent in cancer treatment .
- In Vitro Studies : The compound exhibited potent cytotoxicity against various cancer cell lines, including Huh7 (hepatocellular carcinoma) and Caco-2 (colon cancer), with low micromolar IC50 values .
Enzymatic Inhibition
Tris(dibenzylideneacetone)dipalladium(0) has shown promise in inhibiting Class I PI3-kinase enzymes, which are implicated in cancer cell proliferation and survival. This inhibition can lead to reduced tumor growth and enhanced apoptosis in malignant cells .
Case Studies
Q & A
Q. What is the role of Tris(dibenzylideneacetone)dipalladium(0) (Pd₂(dba)₃) in cross-coupling reactions?
Level: Basic
Answer: Pd₂(dba)₃ serves as a homogeneous Pd(0) catalyst precursor, providing a soluble source of palladium for coupling reactions. The labile dba ligands facilitate ligand displacement, enabling coordination of substrates or ancillary ligands critical for catalytic cycles. It is widely used in Suzuki-Miyaura, Negishi, and Buchwald-Hartwig amination reactions due to its ability to activate aryl halides and promote oxidative addition .
Q. How should Pd₂(dba)₃ be stored to maintain stability?
Level: Basic
Answer: Pd₂(dba)₃ is light-sensitive and should be stored in airtight containers under inert gas (e.g., argon) at ≤15°C in dark conditions. Exposure to moisture or oxygen accelerates decomposition, leading to reduced catalytic activity. Commercial samples often contain chloroform adducts (e.g., Pd₂(dba)₃·CHCl₃), which stabilize the compound during storage .
Q. How does ligand choice influence the catalytic activity of Pd₂(dba)₃ in asymmetric synthesis?
Level: Advanced
Answer: The dba ligands in Pd₂(dba)₃ are readily displaced by stronger donor ligands (e.g., phosphines, N-heterocyclic carbenes), which dictate reaction selectivity and turnover. For example, chiral ligands like BINAP or PHOX induce enantioselectivity in asymmetric allylic alkylation or amination. Ligand-to-palladium ratios and steric/electronic properties must be optimized to suppress off-cycle intermediates and enhance catalytic efficiency .
Q. What factors contribute to variability in Pd₂(dba)₃ catalytic performance across studies?
Level: Advanced
Answer: Key factors include:
- Purity: Commercial Pd₂(dba)₃ purity ranges from >75% (containing dba decomposition byproducts) to >97% (highly active for sensitive reactions) .
- Adducts: Chloroform adducts may require pre-drying to avoid solvent interference .
- Ligand dissociation kinetics: Slow ligand exchange can delay catalytic cycle initiation, necessitating pre-activation with reducing agents or heat .
Contradictory reactivity reports often stem from unaccounted impurities or adducts, emphasizing the need for rigorous batch characterization via NMR or elemental analysis .
Q. How can reaction conditions be optimized for Pd₂(dba)₃ in Buchwald-Hartwig amination?
Level: Advanced
Answer: Optimization involves:
- Ligand selection: Bulky phosphines (e.g., XPhos, SPhos) suppress β-hydride elimination and enhance C–N bond formation .
- Base choice: Strong bases (e.g., Cs₂CO₃) deprotonate amines, while mild bases (K₃PO₄) minimize side reactions .
- Solvent effects: Toluene or dioxane improves solubility of aryl halides and amines.
A representative protocol uses 1.5 mol% Pd₂(dba)₃, 4 mol% SPhos, and K₃PO₄ in toluene at 100°C, achieving >90% yield in aryl amination .
Q. What analytical methods confirm the structure and purity of Pd₂(dba)₃?
Level: Basic
Answer:
- ¹H/¹³C NMR: Identifies dba ligand integrity (e.g., characteristic alkene signals at δ 6.8–7.3 ppm) .
- Elemental analysis: Verifies Pd content (theoretical: ~23.2% Pd; deviations indicate impurities) .
- IR spectroscopy: Confirms carbonyl stretches (~1600 cm⁻¹ for dba ligands) .
- X-ray crystallography: Resolves Pd–alkene bonding geometry and adduct formation .
Q. Why does Pd₂(dba)₃ sometimes require pre-activation in catalytic cycles?
Level: Advanced
Answer: Pre-activation (e.g., heating with ligands or reducing agents) accelerates dba ligand dissociation, generating active Pd(0) species. In situ reduction of Pd(II) impurities (common in aged samples) using Zn or HCO₂Na may also be necessary. Slow ligand exchange kinetics can stall reactions, particularly with electron-deficient substrates .
Q. What safety precautions are essential when handling Pd₂(dba)₃?
Level: Basic
Answer:
- PPE: Nitrile gloves, lab coats, and safety goggles to avoid skin/eye contact (H351 hazard) .
- Ventilation: Use fume hoods to prevent inhalation of fine particles.
- Waste disposal: Collect residues in sealed containers for heavy-metal waste processing .
- Spill management: Avoid dry sweeping; use damp cloths to minimize dust dispersion .
Q. How does Pd₂(dba)₃ compare to other Pd(0) sources (e.g., Pd(PPh₃)₄) in catalytic applications?
Level: Advanced
Answer:
Q. What mechanistic insights explain Pd₂(dba)₃’s efficacy in C–H activation?
Level: Advanced
Answer: Pd₂(dba)₃ facilitates oxidative addition of C–H bonds via a Pd(0)/Pd(II) cycle. The dba ligands stabilize Pd(0), enabling reversible coordination to substrate π-systems. In allylic C–H functionalization, ligand dissociation creates vacant sites for substrate binding, while bulky ligands (e.g., tBuBrettPhos) direct regioselectivity. Kinetic studies show rate-limiting C–H cleavage, with turnover frequencies (TOFs) dependent on ligand electronics .
Properties
IUPAC Name |
1,5-diphenylpenta-1,4-dien-3-one;palladium | |
---|---|---|
Details | Computed by Lexichem TK 2.7.0 (PubChem release 2021.05.07) | |
Source | PubChem | |
URL | https://pubchem.ncbi.nlm.nih.gov | |
Description | Data deposited in or computed by PubChem | |
InChI |
InChI=1S/3C17H14O.2Pd/c3*18-17(13-11-15-7-3-1-4-8-15)14-12-16-9-5-2-6-10-16;;/h3*1-14H;; | |
Details | Computed by InChI 1.0.6 (PubChem release 2021.05.07) | |
Source | PubChem | |
URL | https://pubchem.ncbi.nlm.nih.gov | |
Description | Data deposited in or computed by PubChem | |
InChI Key |
CYPYTURSJDMMMP-UHFFFAOYSA-N | |
Details | Computed by InChI 1.0.6 (PubChem release 2021.05.07) | |
Source | PubChem | |
URL | https://pubchem.ncbi.nlm.nih.gov | |
Description | Data deposited in or computed by PubChem | |
Canonical SMILES |
C1=CC=C(C=C1)C=CC(=O)C=CC2=CC=CC=C2.C1=CC=C(C=C1)C=CC(=O)C=CC2=CC=CC=C2.C1=CC=C(C=C1)C=CC(=O)C=CC2=CC=CC=C2.[Pd].[Pd] | |
Details | Computed by OEChem 2.3.0 (PubChem release 2021.05.07) | |
Source | PubChem | |
URL | https://pubchem.ncbi.nlm.nih.gov | |
Description | Data deposited in or computed by PubChem | |
Molecular Formula |
C51H42O3Pd2 | |
Details | Computed by PubChem 2.1 (PubChem release 2021.05.07) | |
Source | PubChem | |
URL | https://pubchem.ncbi.nlm.nih.gov | |
Description | Data deposited in or computed by PubChem | |
Molecular Weight |
915.7 g/mol | |
Details | Computed by PubChem 2.1 (PubChem release 2021.05.07) | |
Source | PubChem | |
URL | https://pubchem.ncbi.nlm.nih.gov | |
Description | Data deposited in or computed by PubChem | |
Retrosynthesis Analysis
AI-Powered Synthesis Planning: Our tool employs the Template_relevance Pistachio, Template_relevance Bkms_metabolic, Template_relevance Pistachio_ringbreaker, Template_relevance Reaxys, Template_relevance Reaxys_biocatalysis model, leveraging a vast database of chemical reactions to predict feasible synthetic routes.
One-Step Synthesis Focus: Specifically designed for one-step synthesis, it provides concise and direct routes for your target compounds, streamlining the synthesis process.
Accurate Predictions: Utilizing the extensive PISTACHIO, BKMS_METABOLIC, PISTACHIO_RINGBREAKER, REAXYS, REAXYS_BIOCATALYSIS database, our tool offers high-accuracy predictions, reflecting the latest in chemical research and data.
Strategy Settings
Precursor scoring | Relevance Heuristic |
---|---|
Min. plausibility | 0.01 |
Model | Template_relevance |
Template Set | Pistachio/Bkms_metabolic/Pistachio_ringbreaker/Reaxys/Reaxys_biocatalysis |
Top-N result to add to graph | 6 |
Feasible Synthetic Routes
Disclaimer and Information on In-Vitro Research Products
Please be aware that all articles and product information presented on BenchChem are intended solely for informational purposes. The products available for purchase on BenchChem are specifically designed for in-vitro studies, which are conducted outside of living organisms. In-vitro studies, derived from the Latin term "in glass," involve experiments performed in controlled laboratory settings using cells or tissues. It is important to note that these products are not categorized as medicines or drugs, and they have not received approval from the FDA for the prevention, treatment, or cure of any medical condition, ailment, or disease. We must emphasize that any form of bodily introduction of these products into humans or animals is strictly prohibited by law. It is essential to adhere to these guidelines to ensure compliance with legal and ethical standards in research and experimentation.