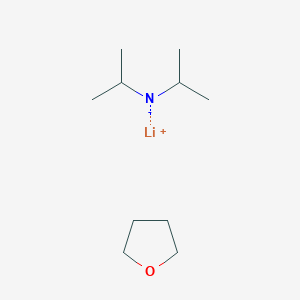
Lithium diisopropylamide mono(tetrahydrofuran)
Overview
Description
Lithium diisopropylamide mono(tetrahydrofuran) is a chemical compound with the molecular formula C10H22LiNO. It is a solution of lithium diisopropylamide in tetrahydrofuran, commonly used as a strong, non-nucleophilic base in organic synthesis. This compound is particularly valued for its ability to deprotonate weakly acidic compounds without attacking carbonyl groups, making it a versatile reagent in various chemical reactions .
Scientific Research Applications
Lithium diisopropylamide mono(tetrahydrofuran) is widely used in scientific research due to its strong basicity and non-nucleophilic nature. Some of its applications include:
Biology: Employed in the preparation of phenylacetic acid components for muscarinic M3 receptor antagonists.
Medicine: Utilized in the synthesis of various pharmaceutical intermediates and active compounds.
Mechanism of Action
Target of Action
Lithium diisopropylamide (commonly abbreviated as LDA) is a strong base . Its primary targets are protons in carbon-hydrogen compounds . It abstracts hydrogen from active carbon , making it a key player in deprotonation reactions .
Mode of Action
LDA interacts with its targets by abstracting a proton from carbon-hydrogen compounds . This interaction results in the formation of carbanion or enolate anions from carbonyl group-containing compounds . The deprotonation process is facilitated by LDA’s non-nucleophilic nature, which prevents it from reacting with other groups in the molecule .
Biochemical Pathways
The primary biochemical pathway affected by LDA is the deprotonation of carbon-hydrogen compounds . This process leads to the generation of carbanion or enolate anions . These anions can then participate in various downstream reactions, such as the formation of carbon-carbon bonds, contributing to the synthesis of complex organic molecules .
Pharmacokinetics
As a strong base, LDA’s pharmacokinetic properties are primarily determined by its reactivity and solubility . It is highly reactive, readily participating in deprotonation reactions . Its good solubility in non-polar organic solvents enhances its bioavailability . It’s important to note that lda is usually generated and observed only in solution .
Result of Action
The action of LDA results in the formation of carbanion or enolate anions from carbonyl group-containing compounds . These anions can then be used in further reactions, such as the formation of carbon-carbon bonds . This makes LDA a valuable tool in organic synthesis .
Action Environment
The action of LDA is influenced by several environmental factors. Its reactivity and efficacy are enhanced in non-polar organic solvents , which also improve its stability . The temperature can also affect the extent of LDA’s aggregation in solution, which in turn can influence its reactivity . Furthermore, LDA is sensitive to moisture and oxygen, which can lead to its decomposition .
Safety and Hazards
Future Directions
Preparation Methods
Synthetic Routes and Reaction Conditions
Lithium diisopropylamide mono(tetrahydrofuran) is typically prepared by reacting diisopropylamine with n-butyllithium in the presence of tetrahydrofuran. The reaction is carried out at low temperatures, usually between 0°C and -78°C, to ensure the stability of the product .
Industrial Production Methods
In industrial settings, the preparation of lithium diisopropylamide mono(tetrahydrofuran) follows similar synthetic routes but on a larger scale. The reaction conditions are carefully controlled to maintain the purity and concentration of the product. The compound is often supplied as a solution in cyclohexane or other non-polar solvents to facilitate its use in various applications .
Chemical Reactions Analysis
Types of Reactions
Lithium diisopropylamide mono(tetrahydrofuran) primarily undergoes deprotonation reactions. It is used to generate carbanions or enolate anions from carbonyl-containing compounds. These anions can then participate in various reactions, including alkylation, acylation, aldol condensation, and α-selenation .
Common Reagents and Conditions
Reagents: Diisopropylamine, n-butyllithium, tetrahydrofuran.
Conditions: Low temperatures (0°C to -78°C), inert atmosphere (usually nitrogen or argon).
Major Products
The major products formed from reactions involving lithium diisopropylamide mono(tetrahydrofuran) include enolates, which can further react to form α,β-unsaturated carbonyl compounds, alkylated ketones, and other derivatives .
Comparison with Similar Compounds
Similar Compounds
Lithium diisopropylamide: A strong base used in similar applications but without the tetrahydrofuran coordination.
Lithium bis(trimethylsilyl)amide: Another strong base with different steric properties.
Potassium tert-butoxide: A strong base used for forming less substituted alkenes in elimination reactions
Uniqueness
Lithium diisopropylamide mono(tetrahydrofuran) is unique due to its coordination with tetrahydrofuran, which enhances its solubility and stability in non-polar solvents. This coordination also allows for more selective deprotonation reactions compared to other bases .
Properties
IUPAC Name |
lithium;di(propan-2-yl)azanide;oxolane | |
---|---|---|
Source | PubChem | |
URL | https://pubchem.ncbi.nlm.nih.gov | |
Description | Data deposited in or computed by PubChem | |
InChI |
InChI=1S/C6H14N.C4H8O.Li/c1-5(2)7-6(3)4;1-2-4-5-3-1;/h5-6H,1-4H3;1-4H2;/q-1;;+1 | |
Source | PubChem | |
URL | https://pubchem.ncbi.nlm.nih.gov | |
Description | Data deposited in or computed by PubChem | |
InChI Key |
YTJXGDYAEOTOCG-UHFFFAOYSA-N | |
Source | PubChem | |
URL | https://pubchem.ncbi.nlm.nih.gov | |
Description | Data deposited in or computed by PubChem | |
Canonical SMILES |
[Li+].CC(C)[N-]C(C)C.C1CCOC1 | |
Source | PubChem | |
URL | https://pubchem.ncbi.nlm.nih.gov | |
Description | Data deposited in or computed by PubChem | |
Molecular Formula |
C10H22LiNO | |
Source | PubChem | |
URL | https://pubchem.ncbi.nlm.nih.gov | |
Description | Data deposited in or computed by PubChem | |
DSSTOX Substance ID |
DTXSID30924467 | |
Record name | Lithium N-(propan-2-yl)propan-2-aminide--oxolane (1/1/1) | |
Source | EPA DSSTox | |
URL | https://comptox.epa.gov/dashboard/DTXSID30924467 | |
Description | DSSTox provides a high quality public chemistry resource for supporting improved predictive toxicology. | |
Molecular Weight |
179.3 g/mol | |
Source | PubChem | |
URL | https://pubchem.ncbi.nlm.nih.gov | |
Description | Data deposited in or computed by PubChem | |
CAS No. |
123333-84-6 | |
Record name | Lithium N-(propan-2-yl)propan-2-aminide--oxolane (1/1/1) | |
Source | EPA DSSTox | |
URL | https://comptox.epa.gov/dashboard/DTXSID30924467 | |
Description | DSSTox provides a high quality public chemistry resource for supporting improved predictive toxicology. | |
Record name | N-(1-Methylethyl)-2-propanamine lithium salt, compd. with Tetrahydrofuran (1:1) | |
Source | European Chemicals Agency (ECHA) | |
URL | https://echa.europa.eu/information-on-chemicals | |
Description | The European Chemicals Agency (ECHA) is an agency of the European Union which is the driving force among regulatory authorities in implementing the EU's groundbreaking chemicals legislation for the benefit of human health and the environment as well as for innovation and competitiveness. | |
Explanation | Use of the information, documents and data from the ECHA website is subject to the terms and conditions of this Legal Notice, and subject to other binding limitations provided for under applicable law, the information, documents and data made available on the ECHA website may be reproduced, distributed and/or used, totally or in part, for non-commercial purposes provided that ECHA is acknowledged as the source: "Source: European Chemicals Agency, http://echa.europa.eu/". Such acknowledgement must be included in each copy of the material. ECHA permits and encourages organisations and individuals to create links to the ECHA website under the following cumulative conditions: Links can only be made to webpages that provide a link to the Legal Notice page. | |
Synthesis routes and methods I
Procedure details
Synthesis routes and methods II
Procedure details
Synthesis routes and methods III
Procedure details
Synthesis routes and methods IV
Procedure details
Retrosynthesis Analysis
AI-Powered Synthesis Planning: Our tool employs the Template_relevance Pistachio, Template_relevance Bkms_metabolic, Template_relevance Pistachio_ringbreaker, Template_relevance Reaxys, Template_relevance Reaxys_biocatalysis model, leveraging a vast database of chemical reactions to predict feasible synthetic routes.
One-Step Synthesis Focus: Specifically designed for one-step synthesis, it provides concise and direct routes for your target compounds, streamlining the synthesis process.
Accurate Predictions: Utilizing the extensive PISTACHIO, BKMS_METABOLIC, PISTACHIO_RINGBREAKER, REAXYS, REAXYS_BIOCATALYSIS database, our tool offers high-accuracy predictions, reflecting the latest in chemical research and data.
Strategy Settings
Precursor scoring | Relevance Heuristic |
---|---|
Min. plausibility | 0.01 |
Model | Template_relevance |
Template Set | Pistachio/Bkms_metabolic/Pistachio_ringbreaker/Reaxys/Reaxys_biocatalysis |
Top-N result to add to graph | 6 |
Feasible Synthetic Routes
Disclaimer and Information on In-Vitro Research Products
Please be aware that all articles and product information presented on BenchChem are intended solely for informational purposes. The products available for purchase on BenchChem are specifically designed for in-vitro studies, which are conducted outside of living organisms. In-vitro studies, derived from the Latin term "in glass," involve experiments performed in controlled laboratory settings using cells or tissues. It is important to note that these products are not categorized as medicines or drugs, and they have not received approval from the FDA for the prevention, treatment, or cure of any medical condition, ailment, or disease. We must emphasize that any form of bodily introduction of these products into humans or animals is strictly prohibited by law. It is essential to adhere to these guidelines to ensure compliance with legal and ethical standards in research and experimentation.