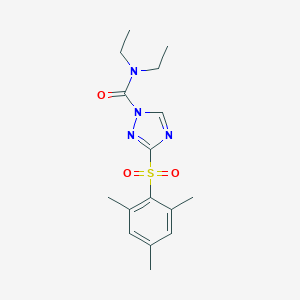
Cafenstrole
Overview
Description
Cafenstrole is a triazole-based herbicide primarily used for the control of annual weeds, particularly grassy weeds, in paddy fields. It is known for its low water solubility and semi-volatility, making it effective in agricultural settings without posing significant risks to non-target organisms . This compound was first registered in Japan in 1997 and has since been used in various countries for its herbicidal properties .
Mechanism of Action
Target of Action
Cafenstrole primarily targets the synthesis of very long-chain fatty acids (VLCFAs) in plants . VLCFAs are crucial for various cellular functions, including cell division .
Mode of Action
This compound acts by inhibiting the synthesis of VLCFAs . This inhibition disrupts cell division, thereby controlling the growth of weeds .
Biochemical Pathways
The primary biochemical pathway affected by this compound is the VLCFA synthesis pathway . By inhibiting this pathway, this compound disrupts the normal growth and development of plants, leading to their eventual death .
Result of Action
The inhibition of VLCFA synthesis by this compound disrupts cell division, leading to the death of the plant . This makes this compound an effective herbicide for controlling the growth of weeds in paddy fields .
Action Environment
This compound is active from pre-emergence to early post-emergence (three-leaf stage) and shows very high selectivity for rice and turf . It has residual activity lasting up to 50 days in paddy fields and 90-150 days in turf . Environmental factors such as soil type, temperature, and moisture levels may influence the action, efficacy, and stability of this compound .
Biochemical Analysis
Biochemical Properties
Cafenstrole acts as a potent inhibitor of the microsomal elongase enzyme . This enzyme plays a crucial role in the biosynthesis of very long-chain fatty acids (VLCFAs) with alkyl chains longer than C18 . The interaction between this compound and this enzyme is crucial in its function as a herbicide.
Cellular Effects
This compound’s inhibition of VLCFA synthesis has significant effects on cellular processes. For instance, it has been observed that defects in VLCFA synthesis can cause cells in the vasculature or in the rib zone of shoot apices to overproliferate . When VLCFA levels decrease, there is an observed increase in the synthesis of the phytohormone cytokinin .
Molecular Mechanism
The molecular mechanism of this compound involves its interaction with the microsomal elongase enzyme, thereby inhibiting the synthesis of VLCFAs . This inhibition disrupts normal cellular processes, particularly those involving cell proliferation .
Metabolic Pathways
This compound’s primary role in metabolic pathways involves the inhibition of VLCFA synthesis . VLCFAs are crucial components of various lipids and are involved in numerous cellular processes. By inhibiting their synthesis, this compound can significantly impact these metabolic pathways.
Preparation Methods
Synthetic Routes and Reaction Conditions: The synthesis of Cafenstrole involves acylation and amination reactions under alkaline conditions. The process typically includes the following steps :
Acylation: The initial step involves the acylation of a triazole ring with a suitable acylating agent.
Amination: The acylated product is then subjected to amination under alkaline conditions to yield this compound.
Industrial Production Methods: Industrial production of this compound follows similar synthetic routes but on a larger scale. The process involves stringent control of reaction conditions to ensure high yield and purity of the final product. The use of advanced chromatographic techniques, such as high-performance liquid chromatography, is common for the purification and validation of this compound .
Chemical Reactions Analysis
Types of Reactions: Cafenstrole undergoes various chemical reactions, including:
Oxidation: this compound can be oxidized to form sulfoxides and sulfones.
Reduction: Reduction reactions can convert this compound to its corresponding amines.
Substitution: Nucleophilic substitution reactions can occur at the triazole ring.
Common Reagents and Conditions:
Oxidation: Common oxidizing agents include hydrogen peroxide and potassium permanganate.
Reduction: Reducing agents such as lithium aluminum hydride are used.
Substitution: Nucleophiles like amines and thiols are employed under basic conditions.
Major Products:
Oxidation: Sulfoxides and sulfones.
Reduction: Amines.
Substitution: Various substituted triazole derivatives.
Scientific Research Applications
Cafenstrole has a wide range of applications in scientific research, including:
Chemistry: Used as a model compound for studying triazole-based herbicides and their interactions with various biological targets.
Comparison with Similar Compounds
Cafenstrole is part of a class of herbicides known as triazole carboxamides. Similar compounds include:
Fentrazamide: Another triazole-based herbicide with similar mechanisms of action.
Mefenacet: An oxyacetamide herbicide that also inhibits very-long-chain fatty acid biosynthesis.
Alachlor: A chloroacetamide herbicide with a broader spectrum of activity but similar target pathways.
Uniqueness: this compound is unique due to its high selectivity for grassy weeds and its low toxicity to non-target organisms, including mammals and honeybees . Its specific inhibition of the microsomal elongase enzyme sets it apart from other herbicides that may have broader or less specific modes of action .
Properties
IUPAC Name |
N,N-diethyl-3-(2,4,6-trimethylphenyl)sulfonyl-1,2,4-triazole-1-carboxamide | |
---|---|---|
Source | PubChem | |
URL | https://pubchem.ncbi.nlm.nih.gov | |
Description | Data deposited in or computed by PubChem | |
InChI |
InChI=1S/C16H22N4O3S/c1-6-19(7-2)16(21)20-10-17-15(18-20)24(22,23)14-12(4)8-11(3)9-13(14)5/h8-10H,6-7H2,1-5H3 | |
Source | PubChem | |
URL | https://pubchem.ncbi.nlm.nih.gov | |
Description | Data deposited in or computed by PubChem | |
InChI Key |
HFEJHAAIJZXXRE-UHFFFAOYSA-N | |
Source | PubChem | |
URL | https://pubchem.ncbi.nlm.nih.gov | |
Description | Data deposited in or computed by PubChem | |
Canonical SMILES |
CCN(CC)C(=O)N1C=NC(=N1)S(=O)(=O)C2=C(C=C(C=C2C)C)C | |
Source | PubChem | |
URL | https://pubchem.ncbi.nlm.nih.gov | |
Description | Data deposited in or computed by PubChem | |
Molecular Formula |
C16H22N4O3S | |
Source | PubChem | |
URL | https://pubchem.ncbi.nlm.nih.gov | |
Description | Data deposited in or computed by PubChem | |
DSSTOX Substance ID |
DTXSID7057949 | |
Record name | Cafenstrole | |
Source | EPA DSSTox | |
URL | https://comptox.epa.gov/dashboard/DTXSID7057949 | |
Description | DSSTox provides a high quality public chemistry resource for supporting improved predictive toxicology. | |
Molecular Weight |
350.4 g/mol | |
Source | PubChem | |
URL | https://pubchem.ncbi.nlm.nih.gov | |
Description | Data deposited in or computed by PubChem | |
CAS No. |
125306-83-4 | |
Record name | Cafenstrole | |
Source | CAS Common Chemistry | |
URL | https://commonchemistry.cas.org/detail?cas_rn=125306-83-4 | |
Description | CAS Common Chemistry is an open community resource for accessing chemical information. Nearly 500,000 chemical substances from CAS REGISTRY cover areas of community interest, including common and frequently regulated chemicals, and those relevant to high school and undergraduate chemistry classes. This chemical information, curated by our expert scientists, is provided in alignment with our mission as a division of the American Chemical Society. | |
Explanation | The data from CAS Common Chemistry is provided under a CC-BY-NC 4.0 license, unless otherwise stated. | |
Record name | Cafenstrole [ISO] | |
Source | ChemIDplus | |
URL | https://pubchem.ncbi.nlm.nih.gov/substance/?source=chemidplus&sourceid=0125306834 | |
Description | ChemIDplus is a free, web search system that provides access to the structure and nomenclature authority files used for the identification of chemical substances cited in National Library of Medicine (NLM) databases, including the TOXNET system. | |
Record name | Cafenstrole | |
Source | EPA DSSTox | |
URL | https://comptox.epa.gov/dashboard/DTXSID7057949 | |
Description | DSSTox provides a high quality public chemistry resource for supporting improved predictive toxicology. | |
Record name | 1H-1,2,4-Triazole-1-carboxamide, N,N-diethyl-3-[(2,4,6-trimethylphenyl)sulfonyl] | |
Source | European Chemicals Agency (ECHA) | |
URL | https://echa.europa.eu/information-on-chemicals | |
Description | The European Chemicals Agency (ECHA) is an agency of the European Union which is the driving force among regulatory authorities in implementing the EU's groundbreaking chemicals legislation for the benefit of human health and the environment as well as for innovation and competitiveness. | |
Explanation | Use of the information, documents and data from the ECHA website is subject to the terms and conditions of this Legal Notice, and subject to other binding limitations provided for under applicable law, the information, documents and data made available on the ECHA website may be reproduced, distributed and/or used, totally or in part, for non-commercial purposes provided that ECHA is acknowledged as the source: "Source: European Chemicals Agency, http://echa.europa.eu/". Such acknowledgement must be included in each copy of the material. ECHA permits and encourages organisations and individuals to create links to the ECHA website under the following cumulative conditions: Links can only be made to webpages that provide a link to the Legal Notice page. | |
Record name | CAFENSTROLE | |
Source | FDA Global Substance Registration System (GSRS) | |
URL | https://gsrs.ncats.nih.gov/ginas/app/beta/substances/II8JP4LY7A | |
Description | The FDA Global Substance Registration System (GSRS) enables the efficient and accurate exchange of information on what substances are in regulated products. Instead of relying on names, which vary across regulatory domains, countries, and regions, the GSRS knowledge base makes it possible for substances to be defined by standardized, scientific descriptions. | |
Explanation | Unless otherwise noted, the contents of the FDA website (www.fda.gov), both text and graphics, are not copyrighted. They are in the public domain and may be republished, reprinted and otherwise used freely by anyone without the need to obtain permission from FDA. Credit to the U.S. Food and Drug Administration as the source is appreciated but not required. | |
Retrosynthesis Analysis
AI-Powered Synthesis Planning: Our tool employs the Template_relevance Pistachio, Template_relevance Bkms_metabolic, Template_relevance Pistachio_ringbreaker, Template_relevance Reaxys, Template_relevance Reaxys_biocatalysis model, leveraging a vast database of chemical reactions to predict feasible synthetic routes.
One-Step Synthesis Focus: Specifically designed for one-step synthesis, it provides concise and direct routes for your target compounds, streamlining the synthesis process.
Accurate Predictions: Utilizing the extensive PISTACHIO, BKMS_METABOLIC, PISTACHIO_RINGBREAKER, REAXYS, REAXYS_BIOCATALYSIS database, our tool offers high-accuracy predictions, reflecting the latest in chemical research and data.
Strategy Settings
Precursor scoring | Relevance Heuristic |
---|---|
Min. plausibility | 0.01 |
Model | Template_relevance |
Template Set | Pistachio/Bkms_metabolic/Pistachio_ringbreaker/Reaxys/Reaxys_biocatalysis |
Top-N result to add to graph | 6 |
Feasible Synthetic Routes
Disclaimer and Information on In-Vitro Research Products
Please be aware that all articles and product information presented on BenchChem are intended solely for informational purposes. The products available for purchase on BenchChem are specifically designed for in-vitro studies, which are conducted outside of living organisms. In-vitro studies, derived from the Latin term "in glass," involve experiments performed in controlled laboratory settings using cells or tissues. It is important to note that these products are not categorized as medicines or drugs, and they have not received approval from the FDA for the prevention, treatment, or cure of any medical condition, ailment, or disease. We must emphasize that any form of bodily introduction of these products into humans or animals is strictly prohibited by law. It is essential to adhere to these guidelines to ensure compliance with legal and ethical standards in research and experimentation.