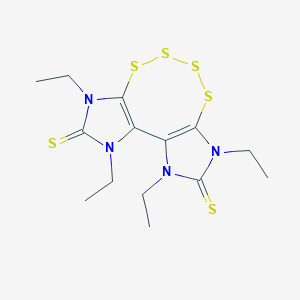
Samarium(III) Ionophore II
- Click on QUICK INQUIRY to receive a quote from our team of experts.
- With the quality product at a COMPETITIVE price, you can focus more on your research.
Overview
Description
Preparation Methods
Synthetic Routes and Reaction Conditions
The synthesis of Samarium(III) Ionophore II involves the reaction of specific organic ligands with samarium ions. One common method includes the copolymerization of samarium(III) with acrylic acid and 4-vinylpyridine in the presence of ethylene glycol dimethacrylate and methyl methacrylate. This process is typically carried out under controlled conditions, including a specific pH, sorption time, and desorption time .
Industrial Production Methods
Industrial production of this compound often involves large-scale polymerization techniques. The process includes the use of ion-exchange methods, solvent extraction, and solid-phase extraction to purify the compound. The final product is characterized using techniques such as Fourier transform infrared spectroscopy, scanning electron microscopy, and energy-dispersive X-ray spectroscopy .
Chemical Reactions Analysis
Types of Reactions
Samarium(III) Ionophore II primarily undergoes complexation reactions with various metal ions. These reactions are crucial for its function as an ionophore. The compound can also participate in oxidation and reduction reactions, depending on the specific reagents and conditions used .
Common Reagents and Conditions
Common reagents used in reactions involving this compound include bidentate ligands such as 1,10-phenanthroline, 2,2’-bipyridine, and neocuproine. These reactions are typically carried out in solvents like dimethyl sulfoxide and under controlled temperature and pH conditions .
Major Products Formed
The major products formed from reactions involving this compound are typically stable complexes with samarium ions. These complexes exhibit unique luminescent properties, making them useful in various applications .
Scientific Research Applications
Samarium(III) Ionophore II has a wide range of scientific research applications:
Chemistry: Used in the selective determination of samarium ions in various samples.
Biology: Employed in bio-assays and imaging techniques due to its luminescent properties.
Medicine: Utilized in radiopharmacy for the treatment of severe pain associated with bone cancer.
Industry: Applied in the purification of samarium-152 stable isotope and in the production of electronic devices
Mechanism of Action
The mechanism of action of Samarium(III) Ionophore II involves the formation of stable complexes with samarium ions. The compound acts as a ligand, binding to the samarium ions through its sulfur and nitrogen atoms. This binding enhances the stability and luminescence of the samarium complexes, making them useful in various applications .
Comparison with Similar Compounds
Similar Compounds
Europium(III) Ionophore: Similar in function but used for europium ions.
Terbium(III) Ionophore: Used for terbium ions and exhibits different luminescent properties.
Neodymium(III) Ionophore: Used for neodymium ions and has distinct applications in optics and electronics.
Uniqueness
Samarium(III) Ionophore II is unique due to its high selectivity for samarium ions and its ability to form stable, luminescent complexes. This makes it particularly valuable in applications requiring precise determination and analysis of samarium ions .
Biological Activity
Samarium(III) Ionophore II (also known as Samarium(III) ionophore) is a compound that has garnered interest due to its potential biological activities, particularly in the context of ion transport and sensor applications. This article explores the biological activity of this compound, focusing on its mechanisms, applications, and relevant research findings.
Overview of this compound
This compound is a synthetic ionophore designed to selectively bind and transport samarium ions (Sm³⁺) across cell membranes. Its chemical structure, characterized by multiple sulfur and nitrogen atoms, facilitates the formation of stable complexes with samarium ions, enhancing their bioavailability and transport efficiency.
Ionophores like this compound function primarily by disrupting ionic homeostasis within cells. They transport specific ions across lipid membranes, which is crucial for various cellular processes, including:
- Membrane Potential Maintenance : By facilitating the movement of Sm³⁺ ions, these ionophores help maintain the electrochemical gradient across cell membranes.
- Cell Signaling : The influx of samarium ions can influence intracellular signaling pathways, potentially affecting cell proliferation and apoptosis.
- Anticancer Activity : Some studies suggest that ionophores can enhance the efficacy of chemotherapeutic agents by altering ion concentrations within cancer cells, making them more susceptible to treatment .
1. Ion Selectivity and Sensor Development
Recent studies have focused on developing sensors utilizing this compound for detecting samarium ions in various environments. For instance:
- Electrode Design : A novel PVC-membrane sensor was developed using 2-{[2-(4-chlorophenyl)-2-oxoethyl]sulfanyl}acetic acid (CSAA), demonstrating high selectivity for Sm³⁺ ions over other cations. The sensor showed a detection limit of 1.0×10−6 M and a linear response range up to 1.0×10−1 M .
Parameter | This Work | Reference 1 | Reference 2 |
---|---|---|---|
Detection Limit | 1.0×10−6 M | 1.0×10−5 M | 1.0×10−5 M |
Linear Range | 1.0×10−6−1.0×10−1 M | 1.0×10−5−1.0×10−1 M | 1.0×10−5−1.0×10−2 M |
Selectivity Coefficient (KMPM) | High | Moderate | Low |
2. Biological Applications
The biological implications of using this compound extend beyond sensor technology:
- Anticancer Properties : Research indicates that ionophores can enhance the cytotoxic effects of anticancer drugs by modulating ion concentrations within tumor cells. For example, Salinomycin, another well-studied ionophore, has shown significant anticancer activity against cancer stem-like cells .
- Toxicity Assessments : Studies on the toxicity of samarium compounds suggest that while samarium ions can be beneficial in low concentrations for certain therapeutic applications, higher concentrations may lead to cytotoxic effects due to disruption of cellular ionic balance .
Case Studies
Several case studies illustrate the practical applications and biological activities associated with this compound:
- Case Study A : Development of a potentiometric sensor for environmental monitoring of samarium levels in water sources demonstrated high accuracy and selectivity, emphasizing its utility in analytical chemistry .
- Case Study B : Investigations into the cellular uptake of samarium ions facilitated by this compound revealed alterations in calcium signaling pathways, suggesting potential applications in targeted drug delivery systems .
Properties
IUPAC Name |
3,5,12,14-tetraethyl-7,8,9,10-tetrathia-3,5,12,14-tetrazatricyclo[9.3.0.02,6]tetradeca-1(11),2(6)-diene-4,13-dithione |
Source
|
---|---|---|
Source | PubChem | |
URL | https://pubchem.ncbi.nlm.nih.gov | |
Description | Data deposited in or computed by PubChem | |
InChI |
InChI=1S/C14H20N4S6/c1-5-15-9-10-12(18(8-4)14(20)16(10)6-2)22-24-23-21-11(9)17(7-3)13(15)19/h5-8H2,1-4H3 |
Source
|
Source | PubChem | |
URL | https://pubchem.ncbi.nlm.nih.gov | |
Description | Data deposited in or computed by PubChem | |
InChI Key |
UCISCPCNYYIDSW-UHFFFAOYSA-N |
Source
|
Source | PubChem | |
URL | https://pubchem.ncbi.nlm.nih.gov | |
Description | Data deposited in or computed by PubChem | |
Canonical SMILES |
CCN1C2=C(N(C1=S)CC)SSSSC3=C2N(C(=S)N3CC)CC |
Source
|
Source | PubChem | |
URL | https://pubchem.ncbi.nlm.nih.gov | |
Description | Data deposited in or computed by PubChem | |
Molecular Formula |
C14H20N4S6 |
Source
|
Source | PubChem | |
URL | https://pubchem.ncbi.nlm.nih.gov | |
Description | Data deposited in or computed by PubChem | |
DSSTOX Substance ID |
DTXSID80327126 |
Source
|
Record name | NSC633113 | |
Source | EPA DSSTox | |
URL | https://comptox.epa.gov/dashboard/DTXSID80327126 | |
Description | DSSTox provides a high quality public chemistry resource for supporting improved predictive toxicology. | |
Molecular Weight |
436.7 g/mol |
Source
|
Source | PubChem | |
URL | https://pubchem.ncbi.nlm.nih.gov | |
Description | Data deposited in or computed by PubChem | |
CAS No. |
120097-53-2 |
Source
|
Record name | NSC633113 | |
Source | EPA DSSTox | |
URL | https://comptox.epa.gov/dashboard/DTXSID80327126 | |
Description | DSSTox provides a high quality public chemistry resource for supporting improved predictive toxicology. | |
Disclaimer and Information on In-Vitro Research Products
Please be aware that all articles and product information presented on BenchChem are intended solely for informational purposes. The products available for purchase on BenchChem are specifically designed for in-vitro studies, which are conducted outside of living organisms. In-vitro studies, derived from the Latin term "in glass," involve experiments performed in controlled laboratory settings using cells or tissues. It is important to note that these products are not categorized as medicines or drugs, and they have not received approval from the FDA for the prevention, treatment, or cure of any medical condition, ailment, or disease. We must emphasize that any form of bodily introduction of these products into humans or animals is strictly prohibited by law. It is essential to adhere to these guidelines to ensure compliance with legal and ethical standards in research and experimentation.