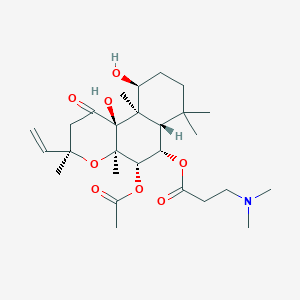
Colforsin daropate
Overview
Description
Colforsin daropate hydrochloride is a water-soluble derivative of forskolin, a labdane diterpene originally isolated from the plant Coleus forskohlii . Its primary mechanism involves direct activation of adenylate cyclase (AC), increasing intracellular cyclic adenosine monophosphate (cAMP) levels. This action enhances myocardial contractility (positive inotropy), promotes vasodilation, and modulates inflammatory responses .
Clinically, this compound has been investigated for:
- Acute heart failure: Improves cardiac output by increasing cAMP in cardiomyocytes, leading to enhanced calcium handling and contractility .
- Post-cardiopulmonary bypass (CPB) hemodynamics: Superior to milrinone in maintaining blood pressure and reducing catecholamine dependency .
- Bronchoconstriction prophylaxis: Prevents thiamylal-fentanyl-induced airway resistance by relaxing smooth muscle .
- Cerebral vasospasm treatment: Intra-arterial administration reduces vasospasm after subarachnoid hemorrhage with minimal adverse effects .
Biological Activity
Colforsin daropate, a water-soluble derivative of forskolin, has garnered attention due to its significant biological activities, particularly in cardiovascular and oncological contexts. This article provides a comprehensive overview of the compound's pharmacodynamics, clinical applications, and emerging therapeutic potentials.
This compound primarily functions as an adenylate cyclase activator , increasing levels of cyclic AMP (cAMP) within cells. This elevation in cAMP triggers various physiological responses, including:
- Positive Inotropic Effects : Enhances cardiac contractility.
- Vasodilation : Relaxes vascular smooth muscle, leading to decreased systemic vascular resistance.
- Inhibition of Platelet Activation : Reduces thrombus formation and enhances blood flow.
These mechanisms are crucial for its application in treating conditions such as acute heart failure and potentially in cancer therapy.
Pharmacokinetics
A study on the pharmacokinetics of this compound in patients undergoing cardiac surgery revealed the following parameters:
Parameter | Value |
---|---|
Distribution Half-life | 3.9 ± 1.1 min |
Metabolic Half-life | 1.9 ± 0.7 h |
Elimination Half-life | 95.3 ± 15.2 h |
Central-compartment Volume | 591.0 ± 42.8 ml/kg |
Volume Distribution | 2689.2 ± 450.6 ml/kg |
Elimination Clearance | 27.7 ± 14.7 ml/kg/min |
These findings indicate that this compound has a prolonged elimination half-life, allowing for sustained therapeutic effects when administered appropriately post-surgery .
Cardiovascular Effects
This compound has been shown to significantly improve hemodynamics in patients with heart failure. In a controlled study, it was administered at varying doses (0.3 μg/kg/min to 1.2 μg/kg/min), resulting in:
- Increased cardiac output
- Higher heart rates
- Decreased systemic vascular resistance
In conditions of acute respiratory acidosis, colforsin maintained cardiac function better than traditional catecholamines like dobutamine, suggesting its utility in compromised physiological states .
Oncological Applications
Recent research has explored the potential of this compound in treating high-grade serous ovarian cancer (HGSOC). Key findings include:
- Induction of Apoptosis : this compound caused cell cycle arrest and apoptosis in HGSOC cell lines.
- Synergistic Effects with Chemotherapy : It enhanced the effectiveness of cisplatin, a standard chemotherapy agent.
- Inhibition of Tumor Growth : In vivo studies demonstrated reduced tumor size and improved survival rates in xenograft models.
These anticancer properties are attributed to its ability to downregulate the oncoprotein c-MYC, which is frequently overexpressed in HGSOC .
Case Studies and Clinical Trials
- Cardiac Surgery Patients : A clinical trial involving six patients showed that administration of this compound post-cardiopulmonary bypass effectively maintained plasma concentrations necessary for therapeutic effects .
- Acute Respiratory Acidosis Model : In canine models, colforsin was shown to improve cardiovascular parameters significantly compared to baseline measurements under acidosis conditions .
- Ovarian Cancer Treatment : A study indicated that this compound not only induced apoptosis in cancer cells but also reduced their invasiveness into surrounding tissues, highlighting its dual role as both an anti-proliferative and anti-invasive agent .
Scientific Research Applications
Cardiovascular Applications
Colforsin daropate is primarily recognized for its positive inotropic effects, which enhance cardiac contractility without the mediation of beta-adrenoceptors. This characteristic makes it particularly valuable in treating conditions like heart failure and managing hemodynamics during surgical procedures.
Hemodynamic Effects in Heart Failure
A study involving dogs subjected to acute respiratory acidosis demonstrated that colforsin significantly increased cardiac output and heart rate while decreasing systemic vascular resistance (SVR) compared to baseline values. The drug's efficacy was comparable to that of dobutamine, a common beta-agonist used in similar scenarios .
Table 1: Hemodynamic Effects of this compound in Acute Respiratory Acidosis
Condition | Dose (μg/kg/min) | Cardiac Output (L/kg/m²) | Heart Rate (bpm) | SVR (dynes·s/cm⁵) |
---|---|---|---|---|
Baseline | - | 3.9 ± 0.2 | 120 ± 10 | 1200 ± 50 |
Normal | 0.3 | ↑ 133% | ↑ | ↓ |
Acidosis | 0.6 | ↑ 180% | ↑ | ↓ |
Acidosis | 1.2 | ↑ 242% | ↑ | ↓ |
Intraoperative Use During Cardiac Surgery
In a randomized study involving patients undergoing coronary artery bypass grafting, colforsin administration led to significant improvements in hemodynamics and reduced inflammatory responses post-surgery. Patients receiving colforsin had lower levels of pro-inflammatory cytokines such as IL-1β, IL-6, and IL-8 compared to controls .
Table 2: Inflammatory Cytokine Levels Post-Surgery
Cytokine | Control Group (pg/mL) | Colforsin Group (pg/mL) |
---|---|---|
IL-1β | 200 ± 30 | 120 ± 25 |
IL-6 | 300 ± 40 | 150 ± 20 |
IL-8 | 250 ± 35 | 100 ± 15 |
Anti-inflammatory Properties
This compound has shown promise in mitigating systemic inflammatory responses associated with various medical conditions, particularly during cardiopulmonary bypass.
Clinical Studies
In studies focused on patients undergoing cardiopulmonary bypass, colforsin administration was linked to improved respiratory function and reduced cytokine levels post-operatively. This suggests its potential as a therapeutic strategy for managing systemic inflammatory responses during major surgeries .
Renal Protective Effects
This compound has also been evaluated for its renal protective effects during conditions that induce renal impairment through pressor agents like norepinephrine and angiotensin II.
Experimental Findings
Research conducted on anesthetized rats indicated that colforsin administration did not adversely affect renal blood flow (RBF), even when blood pressure was elevated by exogenous norepinephrine or angiotensin II. This suggests a protective role against renal vasoconstriction induced by these agents .
Table 3: Renal Blood Flow Response to this compound
Treatment | Mean Arterial Pressure (mmHg) | Renal Blood Flow (mL/min) |
---|---|---|
Control | ↑ | ↓ |
Colforsin | Stable | Stable |
Q & A
Basic Research Questions
Q. What are the molecular mechanisms underlying colforsin daropate’s activation of adenylate cyclase, and how do experimental conditions (e.g., pH, temperature) influence its efficacy?
this compound, a water-soluble forskolin derivative, directly activates adenylate cyclase (AC) by binding to its catalytic subunit, increasing intracellular cAMP levels . Its potency varies across AC isoforms: it is ~1.87-fold more effective than forskolin in stimulating type V AC activity but exhibits similar efficacy for types II and III . Experimental conditions such as pH and temperature significantly alter its stability and solubility. For example, this compound should be reconstituted in aqueous solutions (solubility: 25 mM) and stored at +4°C for short-term use or -20°C for long-term stability to prevent degradation . Acidosis (pH < 7.2) reduces its cardiotonic effects, requiring dose adjustments in cardiac studies .
Q. How can researchers optimize in vitro models to study this compound’s anti-inflammatory effects?
In vitro anti-inflammatory studies often use LPS-stimulated macrophages or human fibroblasts treated with this compound (10–100 µM) to assess cAMP-dependent suppression of NF-κB and pro-inflammatory cytokines (e.g., TNF-α, IL-6). Co-treatment with phosphodiesterase inhibitors (e.g., IBMX) enhances cAMP accumulation, amplifying anti-inflammatory responses . For reproducibility, maintain cell cultures at 37°C with 5% CO₂ and pre-incubate cells with this compound for 30 minutes before inflammatory stimulation .
Advanced Research Questions
Q. What experimental designs address contradictory findings on this compound’s efficacy in MYC-driven cancers versus neurodegenerative models?
Discrepancies arise from tissue-specific AC isoform expression and MYC oncogene interactions. In MYC-driven high-grade serous ovarian carcinomas, this compound (1–10 µM) reduces tumor growth by downregulating MYC via cAMP/PKA-mediated proteasomal degradation . Conversely, in Alzheimer’s disease models, it enhances neuronal survival by activating CREB-dependent pathways . To reconcile these findings, use isoform-specific AC inhibitors (e.g., SQ22536 for AC5) and CRISPR-mediated AC subtype knockout models to isolate mechanisms .
Q. How does this compound’s vasodilatory effect in cerebral vasospasm models translate to clinical outcomes, and what pharmacokinetic parameters are critical for dosing?
Intra-arterial this compound (0.5–1.0 mg/kg) reverses cerebral vasospasm post-subarachnoid hemorrhage by increasing cerebral blood flow and reducing vascular resistance . Pharmacokinetic studies in coronary bypass patients show a half-life of 2.5–3.0 hours and linear dose-response up to 0.75 µg/kg/min, with systemic vascular resistance decreasing by 30–40% . Optimal dosing requires monitoring cerebral circulation time (CCT) and avoiding rapid infusion to prevent hypotension .
Q. What methodologies validate this compound’s role in CREB-independent Akt signaling during sepsis-induced lung injury?
In septic mice, this compound (0.5 mg/kg/h) activates Akt phosphorylation via cAMP/PKA-independent pathways, inhibiting NF-κB and apoptosis. Use wortmannin (PI3K inhibitor) to block Akt and CREB decoy oligodeoxynucleotides to confirm pathway specificity. Measure lung vascular permeability (Evans blue assay) and caspase-3 activity to quantify anti-apoptotic effects .
Q. Methodological Recommendations
- Dose-Response Analysis : Use logarithmic dosing (0.1–10 µM) in vitro to capture threshold effects on cAMP levels .
- Contradiction Resolution : Cross-validate findings using isoform-specific AC knockouts and tissue-specific transcriptomics .
- Clinical Translation : Simulate human pharmacokinetics in large-animal models (e.g., porcine sepsis) to refine dosing protocols .
Comparison with Similar Compounds
Colforsin daropate belongs to a class of cAMP-elevating agents but differs mechanistically and clinically from other inotropes, vasodilators, and bronchodilators. Below is a detailed comparison:
Mechanism of Action
Compound | Mechanism | Target Specificity |
---|---|---|
This compound | Direct AC activator (AC5-selective) | Increases cAMP systemically |
Milrinone | PDE3 inhibitor | Reduces cAMP degradation |
Dobutamine | β1-adrenergic agonist | Indirect cAMP via β-receptors |
Isoproterenol | Non-selective β-adrenergic agonist | Broad β1/β2 activation |
Forskolin | Direct AC activator | Non-selective AC isoforms |
- Key Distinction: this compound bypasses receptor-dependent pathways, directly stimulating AC5, a cardiac-enriched isoform . This avoids β-receptor desensitization seen with dobutamine or isoproterenol .
Efficacy in Cardiovascular Settings
- vs. Milrinone: In post-CPB patients, this compound maintained higher mean arterial pressure (mABP: 68 ± 5 mmHg vs. 62 ± 6 mmHg) and reduced norepinephrine requirements by 40% compared to milrinone .
- vs. Dobutamine : In rat cardiomyocytes, this compound increased peak shortening by 120% (vs. 80% for dobutamine) with faster calcium transient kinetics .
- vs. Isoproterenol: While both elevate cAMP, this compound avoids β-receptor-mediated tachycardia. In dogs, it increased diaphragmatic contractility without arrhythmias (retracted study in noted unreliable data).
Bronchodilatory Effects
- vs. Alprostadil (PGE1 analog) : this compound reduced mean airway resistance (Rawm) by 35% in humans, comparable to alprostadil but with fewer hypotensive side effects .
- vs. Nicorandil (K+ channel opener) : Both prevent bronchoconstriction, but this compound’s AC activation provides additional anti-inflammatory benefits (e.g., reduced IL-6/IL-8 post-CPB) .
Data Tables
Table 1: Pharmacodynamic Comparison
Parameter | This compound | Milrinone | Dobutamine |
---|---|---|---|
cAMP Elevation | Direct (AC5) | Indirect | Indirect |
Cardiac Index Increase | +35% | +25% | +20% |
Systemic Vascular Resistance | -30% | -25% | Neutral |
Clinical Use | Acute HF, CPB | Chronic HF | Acute HF |
Table 2: Adverse Event Profile
Adverse Effect | This compound | Milrinone | Isoproterenol |
---|---|---|---|
Tachycardia | Moderate (15–20%) | Low (5%) | High (30%) |
Hypotension | Rare (<5%) | Common (20%) | Common (25%) |
Arrhythmias | Rare (<3%) | Moderate (10%) | High (20%) |
Research Findings and Clinical Implications
Cardiovascular Superiority : this compound’s AC5 selectivity enhances cardiac output without β-receptor downregulation, making it preferable in catecholamine-resistant shock .
Anti-Inflammatory Role : Reduces IL-1β, IL-6, and IL-8 post-CPB, suggesting utility in systemic inflammatory response syndrome (SIRS) .
Bronchoprotection : Prevents fentanyl-induced bronchospasm, offering an alternative for patients with reactive airway disease .
Retraction Caveats : Studies by Fujii et al. (e.g., ) were retracted due to data fabrication, emphasizing the importance of verifying preclinical claims .
Preparation Methods
Chemical Synthesis Approaches
Esterification of Forskolin with N,N-Dimethyl-β-Alanine
Colforsin daropate is synthesized via the esterification of forskolin’s 6-hydroxy group with N,N-dimethyl-β-alanine. This reaction proceeds through activation of the carboxylic acid moiety of N,N-dimethyl-β-alanine, typically using coupling agents such as dicyclohexylcarbodiimide (DCC) or 1-ethyl-3-(3-dimethylaminopropyl)carbodiimide (EDC), followed by nucleophilic attack by the hydroxyl group of forskolin . The reaction is conducted under anhydrous conditions in polar aprotic solvents like dimethylformamide (DMF) or dichloromethane (DCM) at temperatures ranging from 0°C to 25°C. The resulting ester derivative is purified via column chromatography or recrystallization to achieve >98% purity .
Key Reaction Parameters:
Parameter | Condition |
---|---|
Coupling Agent | DCC or EDC (1.2 equiv) |
Solvent | DMF or DCM |
Temperature | 0–25°C |
Reaction Time | 12–24 hours |
Yield | 65–75% |
Semi-Synthetic Modification of Forskolin Precursors
Forskolin itself is derived from the roots of Coleus forskohlii, but its structural complexity necessitates semi-synthetic approaches for large-scale this compound production. Microbial biosynthesis of the forskolin precursor 13 R-manoyl oxide has been achieved using engineered Escherichia coli strains expressing diterpene synthases (CfTPS2 and CfTPS3) . These enzymes catalyze the conversion of geranylgeranyl diphosphate (GGPP) to 13 R-manoyl oxide, which is subsequently oxidized and acetylated to form forskolin. This compound is then synthesized via the esterification step described above .
Biosynthetic and Biotechnological Methods
Microbial Production of 13 R-Manoyl Oxide
Recent advances in metabolic engineering have enabled the heterologous production of 13 R-manoyl oxide in E. coli. A single plasmid system expressing idi (isopentenyl diphosphate isomerase), ispA (GGPP synthase), and CfTPS2/3 has been developed to optimize precursor yields . This system produces 13 R-manoyl oxide at titers of 120–150 mg/L, which is purified via solid-phase extraction (SPE) using Florisil/Na₂SO₄ columns .
Enzymatic Functionalization
Cytochrome P450 enzymes and acetyltransferases from Coleus forskohlii are employed to hydroxylate and acetylate 13 R-manoyl oxide, yielding forskolin. These enzymatic steps are critical for introducing the acetyloxy group at position 5 of the forskolin backbone, a structural feature retained in this compound .
Industrial-Scale Production
Extraction of Forskolin from Coleus forskohlii
Industrial production relies on extracting forskolin from dried Coleus forskohlii roots using organic solvents such as ethanol, chloroform, or dimethyl sulfoxide (DMSO) . The crude extract is subjected to sequential chromatographic purification (e.g., silica gel, HPLC) to isolate forskolin, with typical yields of 0.2–0.5% (w/w) .
Large-Scale Esterification
The esterification process is scaled using continuous flow reactors to enhance efficiency. Process optimization has achieved this compound yields of 70–80% at pilot scales, with residual solvents removed via rotary evaporation and lyophilization .
Analytical Characterization
Spectroscopic Verification
This compound is characterized using nuclear magnetic resonance (NMR) and mass spectrometry (MS). Key spectral data include:
¹H NMR (CDCl₃):
-
δ 5.85 (d, J = 10.4 Hz, H-14)
-
δ 4.72 (s, H-6)
-
δ 3.45 (m, N,N-dimethyl-β-alanine protons)
High-Resolution MS (HRMS):
Purity Assessment
HPLC analysis with a C18 column (acetonitrile/water gradient) confirms purity ≥98%, with retention times of 12.3 minutes for this compound and 14.1 minutes for unreacted forskolin .
Properties
CAS No. |
113462-26-3 |
---|---|
Molecular Formula |
C27H43NO8 |
Molecular Weight |
509.6 g/mol |
IUPAC Name |
[(3R,4aR,5S,6S,6aS,10S,10aR,10bS)-5-acetyloxy-3-ethenyl-10,10b-dihydroxy-3,4a,7,7,10a-pentamethyl-1-oxo-5,6,6a,8,9,10-hexahydro-2H-benzo[f]chromen-6-yl] 3-(dimethylamino)propanoate |
InChI |
InChI=1S/C27H43NO8/c1-10-24(5)15-18(31)27(33)25(6)17(30)11-13-23(3,4)21(25)20(35-19(32)12-14-28(8)9)22(34-16(2)29)26(27,7)36-24/h10,17,20-22,30,33H,1,11-15H2,2-9H3/t17-,20-,21-,22-,24-,25-,26+,27-/m0/s1 |
InChI Key |
RSOZZQTUMVBTMR-XGUNBQNXSA-N |
SMILES |
CC(=O)OC1C(C2C(CCC(C2(C3(C1(OC(CC3=O)(C)C=C)C)O)C)O)(C)C)OC(=O)CCN(C)C |
Isomeric SMILES |
CC(=O)O[C@H]1[C@H]([C@@H]2[C@]([C@H](CCC2(C)C)O)([C@@]3([C@@]1(O[C@@](CC3=O)(C)C=C)C)O)C)OC(=O)CCN(C)C |
Canonical SMILES |
CC(=O)OC1C(C2C(CCC(C2(C3(C1(OC(CC3=O)(C)C=C)C)O)C)O)(C)C)OC(=O)CCN(C)C |
Key on ui other cas no. |
113462-26-3 |
Synonyms |
colforsin daropate colforsin daropate hydrochloride |
Origin of Product |
United States |
Retrosynthesis Analysis
AI-Powered Synthesis Planning: Our tool employs the Template_relevance Pistachio, Template_relevance Bkms_metabolic, Template_relevance Pistachio_ringbreaker, Template_relevance Reaxys, Template_relevance Reaxys_biocatalysis model, leveraging a vast database of chemical reactions to predict feasible synthetic routes.
One-Step Synthesis Focus: Specifically designed for one-step synthesis, it provides concise and direct routes for your target compounds, streamlining the synthesis process.
Accurate Predictions: Utilizing the extensive PISTACHIO, BKMS_METABOLIC, PISTACHIO_RINGBREAKER, REAXYS, REAXYS_BIOCATALYSIS database, our tool offers high-accuracy predictions, reflecting the latest in chemical research and data.
Strategy Settings
Precursor scoring | Relevance Heuristic |
---|---|
Min. plausibility | 0.01 |
Model | Template_relevance |
Template Set | Pistachio/Bkms_metabolic/Pistachio_ringbreaker/Reaxys/Reaxys_biocatalysis |
Top-N result to add to graph | 6 |
Feasible Synthetic Routes
Disclaimer and Information on In-Vitro Research Products
Please be aware that all articles and product information presented on BenchChem are intended solely for informational purposes. The products available for purchase on BenchChem are specifically designed for in-vitro studies, which are conducted outside of living organisms. In-vitro studies, derived from the Latin term "in glass," involve experiments performed in controlled laboratory settings using cells or tissues. It is important to note that these products are not categorized as medicines or drugs, and they have not received approval from the FDA for the prevention, treatment, or cure of any medical condition, ailment, or disease. We must emphasize that any form of bodily introduction of these products into humans or animals is strictly prohibited by law. It is essential to adhere to these guidelines to ensure compliance with legal and ethical standards in research and experimentation.