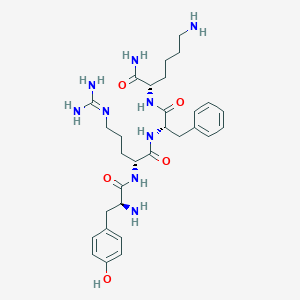
Dalda
Overview
Description
Dalda, chemically known as H-Dmt-D-Arg-Phe-Lys-NH₂ ([Dmt¹]this compound), is a synthetic tetrapeptide opioid agonist with high specificity for the μ-opioid receptor (MOR). Its structure features a modified tyrosine residue (2',6'-dimethyltyrosine, Dmt) at the N-terminal, which enhances receptor binding and metabolic stability . The molecular formula of this compound is C₃₀H₄₅N₉O₅, with a molecular weight of 611.74 g/mol . It exhibits potent antinociceptive effects, prolonged duration of action, and unique bifunctional properties combining μ-opioid agonism and antioxidant activity .
Preparation Methods
Synthetic Routes and Reaction Conditions: Hydrogenation of peanut oil involves the addition of hydrogen gas to the oil in the presence of a catalyst, typically nickel. The reaction is carried out at elevated temperatures (around 140-225°C) and pressures (1-10 atm). The degree of hydrogenation can be controlled to achieve the desired consistency of the final product .
Industrial Production Methods: In industrial settings, hydrogenation is performed in large reactors where peanut oil is mixed with a nickel catalyst and hydrogen gas. The mixture is heated and pressurized to facilitate the hydrogenation reaction. After the reaction is complete, the catalyst is removed, and the hydrogenated oil is refined to remove any impurities .
Chemical Reactions Analysis
Types of Reactions:
Common Reagents and Conditions:
Hydrogen gas: Used as the hydrogen source.
Nickel catalyst: Facilitates the hydrogenation reaction.
Elevated temperature and pressure: Necessary to drive the reaction to completion.
Major Products Formed:
Scientific Research Applications
Pharmacological Properties
Dalda is primarily recognized for its role as a potent mu-opioid receptor (MOR) agonist. Research indicates that modifications to the this compound structure, such as the substitution of amino acids, can significantly enhance its binding affinity and agonist potency:
- Binding Affinity : Studies show that [Dmt1]this compound exhibits a binding affinity in the low nanomolar range, with K_i values as low as 0.143 nM, indicating a strong interaction with the MOR .
- Agonist Potency : The modified peptide [Dmt1]this compound is reported to be 180-fold more potent than its predecessor this compound in inducing G protein activation at the MOR . This enhanced potency is crucial for developing effective analgesic therapies.
Delivery Systems
The effective delivery of this compound analogs to the central nervous system (CNS) poses challenges due to the blood-brain barrier (BBB). Recent advancements in drug delivery systems have shown promise:
- Nanoemulsion Formulation : A study developed an oil-in-water nanoemulsion system encapsulating chemically modified this compound for CNS delivery. This formulation demonstrated efficacy in pain models and was well-tolerated in preliminary safety evaluations . The use of functional magnetic resonance imaging (fMRI) provided quantitative evidence of the analgesic activity of this compound analogs in vivo.
Therapeutic Applications
The analgesic properties of this compound and its analogs position them as potential candidates for pain management therapies:
- Pain Management : The ability of this compound to activate MOR makes it a valuable tool in treating acute and chronic pain conditions. Its high potency and selectivity for MOR suggest it could provide effective pain relief with potentially fewer side effects compared to traditional opioids .
- Research Tool : The fluorescent analogs of this compound have been utilized in binding studies and cellular uptake research, enhancing our understanding of receptor interactions and intracellular distribution . These tools are essential for advancing opioid research and developing new analgesics.
Case Studies and Research Findings
Several studies have documented the efficacy and safety of this compound analogs:
Mechanism of Action
The primary mechanism by which hydrogenated peanut oil exerts its effects is through its increased saturation level. The hydrogenation process reduces the number of double bonds in the fatty acids, making the oil more stable and less prone to oxidation. This increased stability is beneficial in both food and non-food applications, as it extends the shelf life and improves the texture of products .
Comparison with Similar Compounds
Comparison with Similar Compounds
Pharmacological Profile vs. Morphine and DAMGO
Key Findings :
- Potency : Subcutaneous [Dmt¹]this compound is 40–220× more potent than morphine in acute pain models, attributed to enhanced BBB penetration and enzymatic stability .
- Spinal vs. Supraspinal Action : Unlike morphine, [Dmt¹]this compound primarily acts on spinal μ-receptors, with minimal supraspinal activity due to differential receptor variant activation .
- Receptor Binding : [Dmt¹]this compound binds MOR with Kd = 0.199 nM , surpassing DAMGO’s affinity (Kd = 1.2 nM) but with lower κ-receptor selectivity (26:1 vs. DAMGO’s 180:1) .
Binding Affinity and Selectivity vs. Structural Analogues
Modifications at Tyr¹ and Phe³ residues significantly alter receptor interactions:
Compound | μ Receptor (Ki, nM) | δ Receptor (Ki, nM) | κ Receptor (Ki, nM) | Selectivity (μ/δ/κ) |
---|---|---|---|---|
[Dmt¹]this compound | 0.21 | 570 | 5.2 | 10,000:1:25 |
[Tmt¹]this compound | 0.43 | 890 | 6.8 | 2,070:1:16 |
[Emt¹]this compound | 0.55 | 1,200 | 7.5 | 2,180:1:14 |
[Imp³]this compound | 1.2 | 4,670 | 1.1 | 1:3.8:1 |
DAMGO | 1.2 | 180 | 216 | 1:0.15:180 |
Structural Insights :
- Tyr¹ Modifications : Alkyl substituents (e.g., Tmt¹, Emt¹) enhance hydrophobic interactions with MOR but reduce binding affinity by 2–3× compared to [Dmt¹]this compound due to steric hindrance .
- Phe³ Modifications : Substituting Phe³ with bulkier residues (e.g., Imp³) drastically reduces μ-selectivity, increasing κ-receptor affinity by 100× .
Functional Activity and Synergistic Effects
- G Protein Activation : [Dmt¹]this compound and DAMGO are full MOR agonists but partial agonists at κ-receptors in GTPγS assays .
- Antioxidant Activity : The Dmt¹ residue scavenges reactive oxygen species (ROS) with 31.64% DPPH radical inhibition , outperformed by Tmt¹ (34.58%) and Emt¹ (33.51%) analogues .
- Synergy in Neuropathic Pain : [Dmt¹]this compound reverses mechanical allodynia in chronic pain models 15× more effectively than morphine, combining μ-agonism and ROS scavenging .
Pharmacokinetic and Stability Advantages
Property | [Dmt¹]this compound | Morphine | DAMGO |
---|---|---|---|
Metabolic Stability | Resistant to enzymatic degradation | Rapid glucuronidation | Moderate stability |
Placental Transfer | Negligible (<1% maternal-fetal ratio) | High | Not studied |
Clearance | Slow (t₁/₂ = 1.4–1.7 hrs) | Rapid (t₁/₂ = 2–3 hrs) | Moderate |
Therapeutic Implications :
Biological Activity
DALDA (H-Dmt-D-Arg-Phe-Lys-NH2) is a synthetic peptide that acts as a potent μ-opioid receptor (MOR) agonist. It is an analogue of dermorphin, designed to enhance stability and potency in analgesic applications. This article explores the biological activity of this compound, focusing on its pharmacological properties, mechanisms of action, and potential therapeutic applications.
This compound features a modified structure that includes 2′,6′-dimethyltyrosine (Dmt) at the first position. This modification contributes to its high binding affinity and selectivity for the μ-opioid receptor, with a reported Ki value of approximately 0.143 nM . The presence of D-Arg in the second position enhances its enzymatic stability compared to its natural counterpart, dermorphin.
Binding Affinity and Potency:
- Ki Values:
Pharmacological Effects
This compound exhibits significant analgesic effects, particularly in models of neuropathic pain. Studies have demonstrated its ability to reduce thermal hyperalgesia more effectively than morphine, indicating a unique analgesic profile that differs from traditional opioids .
Case Studies:
- Neuropathic Pain Model: In experiments involving neuropathic rats, both ED30 and ED90 doses of [Dmt1]this compound resulted in significantly increased paw withdrawal latencies compared to equivalent doses of morphine .
- Opioid Withdrawal: this compound's effects on opioid withdrawal symptoms were also investigated. It was found that systemic administration of [Dmt1]this compound could partially alleviate withdrawal symptoms, suggesting potential utility in managing opioid dependence .
Mechanistic Insights
The mechanism by which this compound exerts its effects involves several pathways:
- μ-Opioid Receptor Activation: Binding to MOR leads to activation of intracellular signaling pathways that promote analgesia.
- Norepinephrine Uptake Inhibition: this compound also inhibits norepinephrine uptake, contributing to its analgesic effects .
- Endogenous Opioid Release: It has been shown to stimulate the release of endogenous opioids, further enhancing its pain-relieving properties .
Comparative Analysis with Other Opioids
A comparative analysis highlights the unique advantages of this compound over traditional opioids:
Compound | Ki Value (nM) | Potency (vs. Morphine) | Analgesic Effectiveness |
---|---|---|---|
This compound | 0.143 | 3000-fold | High |
[Dmt1]this compound | 0.109 | Higher than this compound | Very High |
Morphine | ~200 | Baseline | Moderate |
Q & A
Basic Research Questions
Q. What methodological considerations are essential when designing experiments to assess Dalda’s μ-opioid receptor selectivity?
- Answer : Experimental design should integrate both in vitro binding assays (e.g., radioligand displacement) and in vivo functional assays (e.g., guinea pig ileum [GPI] for μ-receptor activity and mouse vas deferens [MVD] for δ-receptor activity). Ensure standardized protocols for peptide purity, concentration gradients, and tissue preparation to minimize variability. Cross-validate results using orthogonal methods like cAMP inhibition assays .
Q. How should researchers structure literature reviews to contextualize this compound’s pharmacological profile within existing opioid research?
- Answer : Conduct systematic reviews focusing on:
- Comparative potency of synthetic opioid peptides.
- Structural determinants of receptor selectivity (e.g., Tyr1 modifications).
- Antioxidant mechanisms in neuropathic pain models.
Use databases like PubMed with keywords "this compound analogues," "opioid receptor selectivity," and "dual-function peptides," and prioritize peer-reviewed studies published in the last decade .
Q. What statistical approaches are recommended for analyzing dose-response relationships in this compound’s antinociceptive studies?
- Answer : Apply nonlinear regression models to calculate IC₅₀/EC₅₀ values and compare potency ratios (e.g., GPI vs. MVD assays). Use ANOVA with post-hoc tests (e.g., Tukey’s HSD) to assess significance between analogues. Report confidence intervals and effect sizes to enhance reproducibility .
Q. What criteria determine the selection of appropriate in vitro models for this compound’s receptor selectivity studies?
- Answer : Prioritize tissue-specific models (e.g., GPI for μ-receptor, MVD for δ-receptor) validated in prior literature. Ensure models align with human receptor homology (≥80% sequence similarity). Include control peptides (e.g., DAMGO for μ-receptor specificity) to confirm assay validity .
Advanced Research Questions
Q. How can researchers reconcile discrepancies between in vitro binding affinity data and in vivo functional potency observed in this compound analogues?
- Answer : Investigate pharmacokinetic factors (e.g., blood-brain barrier penetration, metabolic stability) using LC-MS/MS. Perform molecular dynamics simulations to assess peptide-receptor binding kinetics. Compare results with ex vivo autoradiography to validate tissue-level activity .
Q. What strategies validate the dual functionality (opioid agonist and antioxidant) of this compound analogues in neuropathic pain models?
- Answer : Combine receptor-binding assays with antioxidant efficacy tests (e.g., DPPH radical-scavenging assays at 0.085 mM DPPH). Use multivariate regression to correlate substituent electron-donating capacity (e.g., methyl/ethyl groups on Tyr1) with both receptor potency and antioxidant activity (% scavenging). Cross-reference results with in vivo nociception tests (e.g., tail-flick assay) .
Q. What experimental controls are critical when evaluating the antioxidant efficacy of tyrosine-modified this compound derivatives?
- Answer : Include:
- Positive controls (e.g., ascorbic acid) in DPPH assays.
- Negative controls (unmodified this compound) to baseline scavenging activity.
- Solvent controls to rule out assay interference.
Normalize results to peptide concentration (e.g., 0.13 mg/mL) and triplicate runs .
Q. How can multivariate analysis techniques elucidate structure-activity relationships in this compound’s synthetic analogues?
- Answer : Apply QSAR (Quantitative Structure-Activity Relationship) models using descriptors like Hammett constants for substituent electronic effects and LogP for lipophilicity. Validate models with leave-one-out cross-validation and compare predicted vs. experimental IC₅₀ values. Use PCA (Principal Component Analysis) to identify dominant structural drivers of activity .
Q. Data Analysis & Interpretation
Q. How should researchers address conflicting results between this compound’s antioxidant assays and its in vivo neuroprotective efficacy?
- Answer : Conduct dose-ranging studies to identify therapeutic windows. Use correlation matrices to assess relationships between in vitro antioxidant capacity (e.g., % DPPH scavenging) and in vivo outcomes (e.g., reduced allodynia). Consider confounders like oxidative stress biomarkers (e.g., glutathione levels) .
Q. What methods ensure robust reproducibility in synthesizing and characterizing this compound analogues?
- Answer : Document:
- Solid-phase peptide synthesis (SPPS) protocols (e.g., Fmoc chemistry).
- HPLC purity thresholds (>95%).
- Mass spectrometry (MALDI-TOF) for sequence verification.
- Circular dichroism for secondary structure analysis.
Share raw data via repositories like Zenodo with FAIR (Findable, Accessible, Interoperable, Reusable) principles .
Properties
IUPAC Name |
(2S)-6-amino-2-[[(2S)-2-[[(2R)-2-[[(2S)-2-amino-3-(4-hydroxyphenyl)propanoyl]amino]-5-(diaminomethylideneamino)pentanoyl]amino]-3-phenylpropanoyl]amino]hexanamide | |
---|---|---|
Source | PubChem | |
URL | https://pubchem.ncbi.nlm.nih.gov | |
Description | Data deposited in or computed by PubChem | |
InChI |
InChI=1S/C30H45N9O5/c31-15-5-4-9-23(26(33)41)37-29(44)25(18-19-7-2-1-3-8-19)39-28(43)24(10-6-16-36-30(34)35)38-27(42)22(32)17-20-11-13-21(40)14-12-20/h1-3,7-8,11-14,22-25,40H,4-6,9-10,15-18,31-32H2,(H2,33,41)(H,37,44)(H,38,42)(H,39,43)(H4,34,35,36)/t22-,23-,24+,25-/m0/s1 | |
Source | PubChem | |
URL | https://pubchem.ncbi.nlm.nih.gov | |
Description | Data deposited in or computed by PubChem | |
InChI Key |
UEVAHGMTRWGMTB-JBXUNAHCSA-N | |
Source | PubChem | |
URL | https://pubchem.ncbi.nlm.nih.gov | |
Description | Data deposited in or computed by PubChem | |
Canonical SMILES |
C1=CC=C(C=C1)CC(C(=O)NC(CCCCN)C(=O)N)NC(=O)C(CCCN=C(N)N)NC(=O)C(CC2=CC=C(C=C2)O)N | |
Source | PubChem | |
URL | https://pubchem.ncbi.nlm.nih.gov | |
Description | Data deposited in or computed by PubChem | |
Isomeric SMILES |
C1=CC=C(C=C1)C[C@@H](C(=O)N[C@@H](CCCCN)C(=O)N)NC(=O)[C@@H](CCCN=C(N)N)NC(=O)[C@H](CC2=CC=C(C=C2)O)N | |
Source | PubChem | |
URL | https://pubchem.ncbi.nlm.nih.gov | |
Description | Data deposited in or computed by PubChem | |
Molecular Formula |
C30H45N9O5 | |
Source | PubChem | |
URL | https://pubchem.ncbi.nlm.nih.gov | |
Description | Data deposited in or computed by PubChem | |
DSSTOX Substance ID |
DTXSID001045851 | |
Record name | Tyrosyl-arginyl-phenylalanyl-lysinamide | |
Source | EPA DSSTox | |
URL | https://comptox.epa.gov/dashboard/DTXSID001045851 | |
Description | DSSTox provides a high quality public chemistry resource for supporting improved predictive toxicology. | |
Molecular Weight |
611.7 g/mol | |
Source | PubChem | |
URL | https://pubchem.ncbi.nlm.nih.gov | |
Description | Data deposited in or computed by PubChem | |
CAS No. |
68425-36-5, 118476-85-0 | |
Record name | Hydrogenated peanut oil | |
Source | ChemIDplus | |
URL | https://pubchem.ncbi.nlm.nih.gov/substance/?source=chemidplus&sourceid=0068425365 | |
Description | ChemIDplus is a free, web search system that provides access to the structure and nomenclature authority files used for the identification of chemical substances cited in National Library of Medicine (NLM) databases, including the TOXNET system. | |
Record name | Tyrosyl-arginyl-phenylalanyl-lysinamide | |
Source | ChemIDplus | |
URL | https://pubchem.ncbi.nlm.nih.gov/substance/?source=chemidplus&sourceid=0118476850 | |
Description | ChemIDplus is a free, web search system that provides access to the structure and nomenclature authority files used for the identification of chemical substances cited in National Library of Medicine (NLM) databases, including the TOXNET system. | |
Record name | Peanut oil, hydrogenated | |
Source | EPA Chemicals under the TSCA | |
URL | https://www.epa.gov/chemicals-under-tsca | |
Description | EPA Chemicals under the Toxic Substances Control Act (TSCA) collection contains information on chemicals and their regulations under TSCA, including non-confidential content from the TSCA Chemical Substance Inventory and Chemical Data Reporting. | |
Record name | Tyrosyl-arginyl-phenylalanyl-lysinamide | |
Source | EPA DSSTox | |
URL | https://comptox.epa.gov/dashboard/DTXSID001045851 | |
Description | DSSTox provides a high quality public chemistry resource for supporting improved predictive toxicology. | |
Record name | Peanut oil, hydrogenated | |
Source | European Chemicals Agency (ECHA) | |
URL | https://echa.europa.eu/substance-information/-/substanceinfo/100.063.936 | |
Description | The European Chemicals Agency (ECHA) is an agency of the European Union which is the driving force among regulatory authorities in implementing the EU's groundbreaking chemicals legislation for the benefit of human health and the environment as well as for innovation and competitiveness. | |
Explanation | Use of the information, documents and data from the ECHA website is subject to the terms and conditions of this Legal Notice, and subject to other binding limitations provided for under applicable law, the information, documents and data made available on the ECHA website may be reproduced, distributed and/or used, totally or in part, for non-commercial purposes provided that ECHA is acknowledged as the source: "Source: European Chemicals Agency, http://echa.europa.eu/". Such acknowledgement must be included in each copy of the material. ECHA permits and encourages organisations and individuals to create links to the ECHA website under the following cumulative conditions: Links can only be made to webpages that provide a link to the Legal Notice page. | |
Retrosynthesis Analysis
AI-Powered Synthesis Planning: Our tool employs the Template_relevance Pistachio, Template_relevance Bkms_metabolic, Template_relevance Pistachio_ringbreaker, Template_relevance Reaxys, Template_relevance Reaxys_biocatalysis model, leveraging a vast database of chemical reactions to predict feasible synthetic routes.
One-Step Synthesis Focus: Specifically designed for one-step synthesis, it provides concise and direct routes for your target compounds, streamlining the synthesis process.
Accurate Predictions: Utilizing the extensive PISTACHIO, BKMS_METABOLIC, PISTACHIO_RINGBREAKER, REAXYS, REAXYS_BIOCATALYSIS database, our tool offers high-accuracy predictions, reflecting the latest in chemical research and data.
Strategy Settings
Precursor scoring | Relevance Heuristic |
---|---|
Min. plausibility | 0.01 |
Model | Template_relevance |
Template Set | Pistachio/Bkms_metabolic/Pistachio_ringbreaker/Reaxys/Reaxys_biocatalysis |
Top-N result to add to graph | 6 |
Feasible Synthetic Routes
Disclaimer and Information on In-Vitro Research Products
Please be aware that all articles and product information presented on BenchChem are intended solely for informational purposes. The products available for purchase on BenchChem are specifically designed for in-vitro studies, which are conducted outside of living organisms. In-vitro studies, derived from the Latin term "in glass," involve experiments performed in controlled laboratory settings using cells or tissues. It is important to note that these products are not categorized as medicines or drugs, and they have not received approval from the FDA for the prevention, treatment, or cure of any medical condition, ailment, or disease. We must emphasize that any form of bodily introduction of these products into humans or animals is strictly prohibited by law. It is essential to adhere to these guidelines to ensure compliance with legal and ethical standards in research and experimentation.