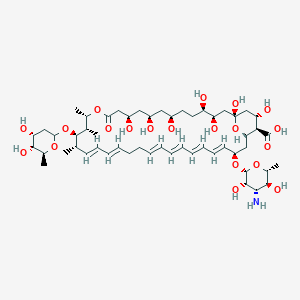
Nystatin A3
Overview
Description
Nystatin A3 is a polyene macrolide antibiotic produced by the bacterium Streptomyces noursei. It is closely related to other nystatin analogues and is known for its potent antifungal properties. This compound is particularly effective against Candida species and other fungi, making it a valuable compound in the treatment of fungal infections .
Scientific Research Applications
Nystatin A3 has a wide range of scientific research applications, including:
Chemistry: Used as a model compound for studying polyene macrolide antibiotics and their chemical modifications.
Biology: Investigated for its interactions with fungal cell membranes and its role in disrupting fungal cell integrity.
Medicine: Employed in the treatment of fungal infections, particularly those caused by Candida species. It is also studied for its potential use in treating other fungal pathogens.
Industry: Utilized in the development of antifungal formulations and as a preservative in various products.
Mechanism of Action
Target of Action
Nystatin A3, like other polyene antifungal drugs, primarily targets ergosterol , a sterol present in the cell membranes of fungi, most notably Candida species . Ergosterol plays a crucial role in maintaining the integrity and fluidity of fungal cell membranes. The interaction between this compound and ergosterol is key to its antifungal activity .
Mode of Action
This compound exerts its antifungal effects by disrupting the fungal cell membrane. It acts as a channel-forming ionophore , creating pores in the fungal plasma membrane . The formation of these pores changes the membrane’s permeability, allowing for leakage of intracellular contents, which ultimately leads to cell death . This interaction is driven by the presence of highly ordered membrane domains capable of stabilizing the Nystatin oligomers .
Biochemical Pathways
The primary antifungal mechanism of Nystatin involves the formation of transmembrane channels that cause the leakage of cellular K+ and Mg2+, eventually leading to the death of the fungal cells . The interactions between Nystatin molecules and ergosterol seem to occur through the conjugated double bonds of the Nystatin molecules .
Pharmacokinetics
This limits its efficacy to the treatment/prevention of cutaneous, mucocutaneous, and gastrointestinal fungal infections . The compound’s ADME (Absorption, Distribution, Metabolism, and Excretion) properties significantly impact its bioavailability and therapeutic effectiveness.
Result of Action
The result of this compound’s action at the molecular and cellular level is the disruption of the fungal cell membrane, leading to cell death . This is achieved through the formation of pores in the membrane, which cause leakage of intracellular contents . In addition, Nystatin-induced pore formation is accompanied by strong Nystatin-induced membrane reorganization that depends on membrane lipid composition .
Action Environment
The action of this compound is influenced by the biophysical properties and lipid composition of the membrane . For instance, in membranes enriched in a gel-phase forming phospholipid, Nystatin incorporates within the phospholipid-enriched gel domains, where it forms pores able to expand the gel domains . In contrast, in membranes enriched in gel domain forming sphingolipids, Nystatin-induced pore formation occurs through the destabilization of the gel phase .
Safety and Hazards
Nystatin A3, like other nystatins, can cause hypersensitivity reactions including rash, pruritus, and anaphylactoid reactions . It may also cause adverse reproductive effects, based on experimental data . The chief hazard associated with exposure during normal use and handling is the potential for irritation of contaminated skin .
Future Directions
Nystatin A3 is a potent antifungal drug with significant toxicity in mammalian organisms . In order to develop a non-toxic and more effective this compound formulation, its molecular mechanism of action at the cell membrane needs to be better understood . Future research may focus on understanding the complex mechanism of action of this compound and developing safer and more effective formulations .
Biochemical Analysis
Biochemical Properties
Nystatin A3 interacts with various biomolecules, primarily membrane sterols, to exert its antifungal effects . It forms aqueous pores in the fungal cell membrane, leading to leakage of intracellular contents and disruption of electrochemical gradients . This interaction is driven by the presence of highly ordered membrane domains capable of stabilizing the this compound oligomers .
Cellular Effects
This compound has significant effects on various types of cells and cellular processes. It influences cell function by disrupting the integrity of the cell membrane, leading to leakage of intracellular contents . This disruption can affect cell signaling pathways, gene expression, and cellular metabolism .
Molecular Mechanism
The mechanism of action of this compound is complex and involves several steps at the molecular level. It forms and stabilizes aqueous pores in the fungal cell membrane, which are associated with its cytotoxicity . This pore formation is accompanied by strong this compound-induced membrane reorganization that depends on membrane lipid composition .
Temporal Effects in Laboratory Settings
The effects of this compound over time in laboratory settings have been observed in studies. For instance, a study showed that this compound exhibited higher therapeutic efficacy over time in white mice with generalized candidiasis .
Metabolic Pathways
This compound is involved in several metabolic pathways. It interacts with enzymes and cofactors, particularly those involved in the synthesis and regulation of membrane sterols
Transport and Distribution
The transport and distribution of this compound within cells and tissues are largely dependent on its interaction with membrane sterols . It is believed to incorporate within phospholipid-enriched gel domains, where it forms pores .
Subcellular Localization
The subcellular localization of this compound is primarily at the cell membrane, where it interacts with membrane sterols to form pores This localization is crucial for its antifungal activity
Preparation Methods
Synthetic Routes and Reaction Conditions
Nystatin A3 is typically produced through fermentation processes involving Streptomyces noursei. The bacterium is cultured under specific conditions that promote the production of nystatin compounds. The fermentation broth is then subjected to extraction and purification processes to isolate this compound .
Industrial Production Methods
Industrial production of this compound involves large-scale fermentation using optimized strains of Streptomyces noursei. The fermentation process is carefully controlled to maximize yield and purity. After fermentation, the compound is extracted using organic solvents and purified through various chromatographic techniques .
Chemical Reactions Analysis
Types of Reactions
Nystatin A3 undergoes several types of chemical reactions, including:
Oxidation: this compound can be oxidized under specific conditions, leading to the formation of various oxidized derivatives.
Reduction: Reduction reactions can modify the polyene structure of this compound, potentially altering its antifungal activity.
Substitution: Substitution reactions involving the hydroxyl groups of this compound can lead to the formation of glycosylated derivatives.
Common Reagents and Conditions
Oxidation: Common oxidizing agents include potassium permanganate and hydrogen peroxide.
Reduction: Reducing agents such as sodium borohydride and lithium aluminum hydride are used.
Substitution: Glycosylation reactions often involve glycosyl donors and catalysts under mild conditions.
Major Products Formed
The major products formed from these reactions include various glycosylated and oxidized derivatives of this compound, which may exhibit different biological activities .
Comparison with Similar Compounds
Similar Compounds
Nystatin A1: Another polyene macrolide antibiotic with similar antifungal properties but differing in its sugar moiety.
Amphotericin B: A related polyene macrolide with a broader spectrum of activity but higher toxicity.
Polyfungin B: A compound with better efficacy against certain fungi compared to Nystatin A3.
Uniqueness
This compound is unique due to its specific glycosylation pattern, which influences its solubility and biological activity. Its reduced toxicity compared to other polyene macrolides makes it a valuable alternative in antifungal therapy .
Properties
IUPAC Name |
33-(4-amino-3,5-dihydroxy-6-methyloxan-2-yl)oxy-17-(4,5-dihydroxy-6-methyloxan-2-yl)oxy-1,3,4,7,9,11,37-heptahydroxy-15,16,18-trimethyl-13-oxo-14,39-dioxabicyclo[33.3.1]nonatriaconta-19,21,25,27,29,31-hexaene-36-carboxylic acid | |
---|---|---|
Details | Computed by Lexichem TK 2.7.0 (PubChem release 2021.05.07) | |
Source | PubChem | |
URL | https://pubchem.ncbi.nlm.nih.gov | |
Description | Data deposited in or computed by PubChem | |
InChI |
InChI=1S/C53H85NO20/c1-29-18-16-14-12-10-8-6-7-9-11-13-15-17-19-37(72-52-49(65)46(54)48(64)33(5)71-52)25-42-45(51(66)67)41(61)28-53(68,74-42)27-40(60)38(58)21-20-34(55)22-35(56)23-36(57)24-43(62)69-31(3)30(2)50(29)73-44-26-39(59)47(63)32(4)70-44/h6-7,9,11-19,29-42,44-50,52,55-61,63-65,68H,8,10,20-28,54H2,1-5H3,(H,66,67) | |
Details | Computed by InChI 1.0.6 (PubChem release 2021.05.07) | |
Source | PubChem | |
URL | https://pubchem.ncbi.nlm.nih.gov | |
Description | Data deposited in or computed by PubChem | |
InChI Key |
IKYMLQOHQLVORI-UHFFFAOYSA-N | |
Details | Computed by InChI 1.0.6 (PubChem release 2021.05.07) | |
Source | PubChem | |
URL | https://pubchem.ncbi.nlm.nih.gov | |
Description | Data deposited in or computed by PubChem | |
Canonical SMILES |
CC1C=CC=CCCC=CC=CC=CC=CC(CC2C(C(CC(O2)(CC(C(CCC(CC(CC(CC(=O)OC(C(C1OC3CC(C(C(O3)C)O)O)C)C)O)O)O)O)O)O)O)C(=O)O)OC4C(C(C(C(O4)C)O)N)O | |
Details | Computed by OEChem 2.3.0 (PubChem release 2021.05.07) | |
Source | PubChem | |
URL | https://pubchem.ncbi.nlm.nih.gov | |
Description | Data deposited in or computed by PubChem | |
Molecular Formula |
C53H85NO20 | |
Details | Computed by PubChem 2.1 (PubChem release 2021.05.07) | |
Source | PubChem | |
URL | https://pubchem.ncbi.nlm.nih.gov | |
Description | Data deposited in or computed by PubChem | |
DSSTOX Substance ID |
DTXSID80212205 | |
Record name | Nystatin A3 | |
Source | EPA DSSTox | |
URL | https://comptox.epa.gov/dashboard/DTXSID80212205 | |
Description | DSSTox provides a high quality public chemistry resource for supporting improved predictive toxicology. | |
Molecular Weight |
1056.2 g/mol | |
Details | Computed by PubChem 2.1 (PubChem release 2021.05.07) | |
Source | PubChem | |
URL | https://pubchem.ncbi.nlm.nih.gov | |
Description | Data deposited in or computed by PubChem | |
CAS No. |
62997-67-5 | |
Record name | Nystatin A3 | |
Source | EPA DSSTox | |
URL | https://comptox.epa.gov/dashboard/DTXSID80212205 | |
Description | DSSTox provides a high quality public chemistry resource for supporting improved predictive toxicology. | |
Q1: Can the glycosylation pattern of Nystatin A3 be modified to generate novel antifungal agents?
A: Yes, research suggests that modifying the glycosylation of polyene macrolides like this compound holds promise for developing new antifungal drugs []. Increasing the extent of glycosylation through enzymatic or chemical methods could potentially reduce toxicity while maintaining or improving antifungal activity. This approach is particularly relevant for addressing the side effects associated with existing polyene antifungals.
Q2: What are the implications of identifying novel sugar components in polyene macrolides like this compound?
A: Discovering a novel sugar component like 2,6-dideoxy-L-ribohexopyranose in this compound [] broadens our understanding of polyene macrolide biosynthesis and structural diversity. This finding suggests the existence of specialized glycosyltransferases in the producing organism, opening avenues for bioengineering efforts to generate new polyene analogues with tailored sugar moieties for improved pharmacological properties.
Disclaimer and Information on In-Vitro Research Products
Please be aware that all articles and product information presented on BenchChem are intended solely for informational purposes. The products available for purchase on BenchChem are specifically designed for in-vitro studies, which are conducted outside of living organisms. In-vitro studies, derived from the Latin term "in glass," involve experiments performed in controlled laboratory settings using cells or tissues. It is important to note that these products are not categorized as medicines or drugs, and they have not received approval from the FDA for the prevention, treatment, or cure of any medical condition, ailment, or disease. We must emphasize that any form of bodily introduction of these products into humans or animals is strictly prohibited by law. It is essential to adhere to these guidelines to ensure compliance with legal and ethical standards in research and experimentation.