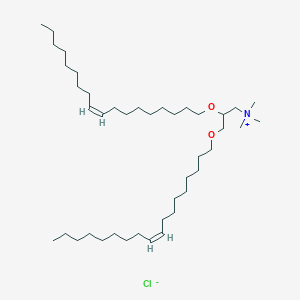
Dotma
Overview
Description
N-[1-(2,3-dioleyloxy)propyl]-N,N,N-trimethylammonium chloride (DOTMA) is a cationic lipid first synthesized in 1987 by Felgner et al. for non-viral gene delivery . Its structure comprises:
- Two oleyl chains linked via ether bonds to a glycerol backbone.
- A quaternary ammonium head group (trimethylammonium) providing strong cationic charge.
- A chloride counterion stabilizing the complexation with nucleic acids .
This compound forms stable complexes (lipoplexes) with DNA/RNA through electrostatic interactions, achieving near 100% nucleic acid encapsulation . It exhibits high transfection efficiency in diverse cell types, including mesenchymal stem cells (MSCs), alveolar cells, and cardiomyocytes . Notably, this compound-based formulations demonstrate lower cytotoxicity compared to commercial reagents like Lipofectamine® .
Preparation Methods
Synthetic Routes and Reaction Conditions
The synthesis of N-[1-(2,3-Dioleyloxy)propyl]-N,N,N-trimethylammonium chloride involves the reaction of N,N,N-trimethylammonium chloride with 1-(2,3-dioleyloxy)propyl chloride. The reaction typically occurs in an organic solvent such as chloroform or dichloromethane under reflux conditions. The product is then purified through column chromatography to obtain the pure compound .
Industrial Production Methods
In industrial settings, the production of N-[1-(2,3-Dioleyloxy)propyl]-N,N,N-trimethylammonium chloride follows a similar synthetic route but on a larger scale. The process involves the use of large reactors and continuous flow systems to ensure high yield and purity. The final product is often subjected to rigorous quality control measures to meet the standards required for biomedical applications .
Chemical Reactions Analysis
Types of Reactions
N-[1-(2,3-Dioleyloxy)propyl]-N,N,N-trimethylammonium chloride primarily undergoes substitution reactions due to the presence of the trimethylammonium group. It can also participate in complexation reactions with nucleic acids, forming stable lipid-DNA complexes .
Common Reagents and Conditions
Substitution Reactions: Typically involve nucleophiles such as hydroxide ions or amines.
Complexation Reactions: Involve the use of plasmid DNA or RNA under aqueous conditions.
Major Products
Substitution Reactions: Yield substituted ammonium compounds.
Complexation Reactions: Form lipid-nucleic acid complexes, which are essential for gene delivery applications.
Scientific Research Applications
Gene Transfection
Mechanism of Action:
DOTMA acts as a non-viral vector that enhances the cellular uptake of nucleic acids. The positive charge on this compound facilitates interactions with negatively charged DNA or RNA, promoting the formation of lipoplexes that can efficiently fuse with cell membranes.
Case Studies:
- In Vivo Studies: Research has demonstrated that this compound-lipid formulations significantly improve transfection efficiency in various cell types. For instance, studies using a CMV-driven expression system showed enhanced luciferase gene expression in mouse models when using this compound compared to other cationic lipids like DOTAP .
- Clinical Relevance: this compound has been utilized in mRNA-based therapies, particularly in cancer immunotherapy. Lipid nanoparticles (LNPs) incorporating this compound have shown promise in delivering mRNA effectively to induce protein expression in target cells .
Liposomal Drug Delivery Systems
Advantages:
Liposomal formulations using this compound exhibit several benefits:
- Biocompatibility: They are less immunogenic compared to conventional drug delivery systems.
- Enhanced Stability: this compound contributes to the stability of liposomes, allowing for prolonged circulation in the bloodstream and improved drug bioavailability .
Data Table: Efficacy Comparison of Liposomal Formulations
Formulation Type | Transfection Efficiency | Stability | Cytotoxicity |
---|---|---|---|
This compound-Liposomes | High | Stable | Low |
DOTAP-Liposomes | Moderate | Moderate | Moderate |
Applications in Cancer Therapy
Mechanism:
this compound is integral in developing lipid nanoparticles for delivering therapeutic agents directly to tumor sites. The ability of these nanoparticles to encapsulate hydrophilic and hydrophobic drugs enhances treatment efficacy while minimizing systemic side effects.
Case Studies:
- Prostate Cancer Therapy: A study demonstrated that dual-modified siRNA-loaded liposomes containing this compound effectively targeted prostate cancer cells, leading to significant tumor reduction .
- Immunotherapy Approaches: Research indicates that mRNA-loaded LNPs utilizing this compound can stimulate robust immune responses against tumors, showcasing its potential in cancer vaccines .
Structural Characteristics and Optimization
Research Findings:
The structural features of this compound play a crucial role in its function:
- Cationic Head Group: The arrangement of the cationic head group relative to the hydrophobic chains is essential for maximizing transfection efficiency.
- Linkage Bonds: The presence of ether bonds instead of ester bonds contributes to the stability and efficacy of this compound in vivo .
Data Table: Structure-Activity Relationship
Structural Feature | Impact on Transfection Efficiency |
---|---|
Ether Bond Presence | Increased |
Cationic Head Group Position | Optimal Interaction |
Length of Aliphatic Chains | Variable Impact |
Mechanism of Action
The mechanism of action of N-[1-(2,3-Dioleyloxy)propyl]-N,N,N-trimethylammonium chloride involves the formation of liposomes that encapsulate nucleic acids. These liposomes interact with the cell membrane, facilitating the entry of the nucleic acids into the cell. The positively charged trimethylammonium group interacts with the negatively charged cell membrane, enhancing the uptake of the liposome-DNA complex .
Comparison with Similar Compounds
Structural Comparisons
Key structural differences between DOTMA and analogous cationic lipids dictate their physicochemical and biological behaviors (Table 1).
Table 1: Structural Comparison of this compound and Similar Compounds
Compound | Fatty Acid Chains | Head Group | Counterion | Key Structural Features |
---|---|---|---|---|
This compound | 2,3-dioleyloxy (C18:1) | Quaternary ammonium | Cl⁻ | Ether linkages, glycerol backbone |
DOTAP | 1,2-dioleoyl (C18:1) | Quaternary ammonium | Br⁻ | Ester linkages, glycerol backbone |
DC-Chol | Cholesteryl | Tertiary amine (carbamate) | Cl⁻ | Cholesterol anchor, biodegradable carbamate |
DDAB | Dimethyldioctadecyl | Quaternary ammonium | Br⁻ | Double branched alkyl chains |
DODMA | 1,2-dioleoyloxy | Tertiary amine | Cl⁻ | Ester linkages, pH-sensitive amine |
Physicochemical Properties
Complexation and Stability
- This compound: Forms smaller, more stable nioplexes (120–190 nm) with DNA/RNA compared to DOTAP and DC-Chol.
- DOTAP : Similar cationic charge density but forms larger complexes due to ester linkages, reducing nucleic acid protection efficiency .
Table 2: Physicochemical Performance
Compound | Nioplex Size (nm) | Zeta Potential (mV) | DNA Protection Efficiency |
---|---|---|---|
This compound | 122–190 | Up to +35.6 | High (SC/OC bands retained) |
DOTAP | 150–250 | Up to +30.0 | Moderate |
DC-Chol | 200–300 | +15–20 | Low |
DDAB | 180–220 | +25–30 | Moderate |
Transfection Efficiency
- This compound: Achieves 80% luciferase gene knockdown at 100 nM siRNA, outperforming DOTAP and DC-Chol by 10-fold in MSCs . β-galactosidase activity in MSCs is comparable to Lipofectamine® but with 66–69% cell viability vs. Lipofectamine®’s 25% .
- DOTAP : Requires higher doses for equivalent efficacy, increasing cytotoxicity .
- DC-Chol : Only 20% HER2 gene silencing at 0.5 μg siRNA/well .
Cytotoxicity
- This compound : Low cytotoxicity due to optimized charge density and oleyl chain fluidity .
- DOTAP : Higher toxicity attributed to bromide counterion and rigid ester linkages .
- DODMA : pH-sensitive tertiary amine reduces long-term toxicity but limits serum stability .
Table 3: Transfection Efficiency and Cytotoxicity
Compound | Transfection Efficiency (%) | Cell Viability (%) | Key Applications |
---|---|---|---|
This compound | 80–100 | 66–69 | mRNA delivery, stem cells |
DOTAP | 50–70 | 40–50 | DNA transfection |
DC-Chol | 20–30 | 60–70 | Low-efficiency siRNA |
DODMA | 60–80 | 70–80 | pH-sensitive RNA delivery |
Environmental Adaptability
- Acidic Conditions : this compound remains stable due to chloride counterion and ether linkages, while DOTAP degrades faster .
- Serum Stability : this compound’s oleyl chains enhance membrane fusion, improving endosomal escape compared to DDAB and DC-Chol .
Advantages of this compound
- High Nucleic Acid Encapsulation : 100% DNA/RNA entrapment via electrostatic interactions .
- Broad Cell Compatibility : Effective in hard-to-transfect cells (e.g., MSCs, cardiomyocytes) .
- Low Cytotoxicity : Superior safety profile compared to DOTAP and Lipofectamine® .
Limitations
Biological Activity
Introduction
DOTMA (N-[1-(2,3-dioleyloxy)propyl]-N,N,N-trimethylammonium chloride) is a cationic lipid widely recognized for its role in gene delivery systems, particularly in non-viral transfection methods. Its structural properties contribute significantly to its biological activity, making it a subject of extensive research in the fields of molecular biology and gene therapy.
Overview of this compound
This compound is characterized by a cationic head group linked to hydrophobic aliphatic chains. The structural features that enhance its transfection efficacy include:
- Cationic Head Group : This positively charged component facilitates electrostatic interactions with negatively charged DNA.
- Hydrophobic Chains : These chains promote the formation of lipid-DNA complexes that can traverse cellular membranes.
- Ether Linkage : The ether bond connecting the aliphatic chains to the backbone is crucial for maintaining the integrity of the lipid structure during transfection.
Structure-Activity Relationship
Research has demonstrated that the effectiveness of this compound in transfection is influenced by:
- The relative positioning of the cationic head group and hydrophobic chains.
- The presence of paired oleyl chains , which serve as a robust hydrophobic anchor.
- The overall structural configuration that allows for optimal interaction with DNA and cellular components upon administration .
Transfection Efficacy
This compound has been shown to facilitate high levels of gene transfer in various cell types. In vivo studies using mouse models have indicated that this compound can achieve significant transfection efficiency, particularly when administered via intravenous routes. A notable study reported up to 65% transfection efficiency in certain cell lines, showcasing its potential for therapeutic applications .
Table 1: Transfection Efficiency of this compound in Various Studies
Study Reference | Cell Line | Transfection Efficiency (%) | Administration Route |
---|---|---|---|
Ren et al. (2000) | Mouse liver cells | 70 | Intravenous |
Science.gov (2018) | Saos-2, MG63 | 65 | Non-viral system |
PubMed (2004) | Primary human cells | 90 | Polysaccharide capsules |
Interaction with DNA
This compound interacts spontaneously with DNA to form stable lipid-DNA complexes. This interaction is critical for effective cellular uptake and subsequent gene expression. The formation of these complexes is facilitated by the cationic nature of this compound, which allows it to condense DNA into smaller particles that can be easily internalized by cells .
Toxicity Profile
While this compound is effective as a transfection agent, studies have also focused on its toxicity profile. Compared to viral vectors, this compound exhibits lower cytotoxicity, making it a favorable option for gene therapy applications. However, careful consideration must be given to dosage and formulation to minimize any potential adverse effects .
Gene Therapy Research
Several case studies have highlighted the application of this compound in gene therapy:
- Lung Cancer Treatment : A study utilized this compound-based lipoplexes to deliver microRNA-29b, demonstrating significant therapeutic effects against lung cancer cells .
- Osteosarcoma Models : Research involving continuous human osteosarcoma cell lines showed successful transfection using this compound, leading to expression of therapeutic genes such as GFP and LacZ .
Future Directions
The ongoing research into optimizing this compound formulations continues to explore its potential in various therapeutic contexts, including:
- Enhancing delivery systems for RNA interference (RNAi).
- Developing targeted delivery mechanisms for specific cell types.
- Investigating combination therapies with other treatment modalities.
Q & A
Basic Research Questions
Q. How to formulate a research question for investigating Dotma's biochemical mechanisms?
A robust research question must address a literature gap, be concise, and align with theoretical frameworks. Begin by reviewing existing studies to identify unresolved hypotheses (e.g., this compound’s interaction with lipid bilayers). Use the FINER criteria (Feasible, Interesting, Novel, Ethical, Relevant) to refine the scope. For example: "How does this compound’s molecular structure influence its efficacy in gene delivery under varying pH conditions?" This question is hypothesis-driven, avoids dichotomous answers, and requires synthesis of structural biology and biophysical data .
Q. What experimental designs are suitable for initial mechanistic studies of this compound?
Pre-experimental or quasi-experimental designs are ideal for exploratory research. For instance, a pretest-posttest control group design (Table 1) can compare this compound’s transfection efficiency against traditional vectors. Include variables like concentration gradients, temperature, and cellular uptake rates. Use factorial designs to test multiple independent variables (e.g., pH and ionic strength) simultaneously .
Group | Treatment | Measurement Metrics |
---|---|---|
Experimental | This compound + Target Cells (pH 7.4) | Transfection Efficiency, Cytotoxicity |
Control | Lipofectamine + Target Cells | Same as Experimental |
Q. How to ensure data validity in this compound-related experiments?
Implement triangulation by combining quantitative (e.g., fluorescence assays) and qualitative (e.g., electron microscopy) methods. Use blinding during data collection to reduce bias. Replicate experiments across independent labs and apply statistical tools (ANOVA, t-tests) to confirm reproducibility. Document protocols in alignment with FAIR principles (Findable, Accessible, Interoperable, Reusable) .
Advanced Research Questions
Q. How to resolve contradictions in published data on this compound’s stability in serum-containing media?
Conduct a meta-analysis of existing datasets to identify confounding variables (e.g., serum concentration, incubation time). Use sensitivity analysis to isolate factors causing disparities. For example, if Study A reports 80% stability (10% serum, 1 hour) and Study B shows 50% (20% serum, 2 hours), design a follow-up experiment varying these parameters systematically. Apply multivariate regression to model interactions .
Q. What methodologies enable integration of multi-omics data to study this compound’s cellular pathways?
Combine transcriptomic (RNA-seq), proteomic (LC-MS), and metabolomic (NMR) datasets using network analysis tools (Cytoscape, STRING). Apply machine learning (e.g., Random Forest) to identify key nodes in this compound-induced pathways. Validate findings with CRISPR-Cas9 knockout models to confirm mechanistic links .
Q. How to design longitudinal studies assessing this compound’s long-term biocompatibility?
Use a cohort study design with staggered observation periods (e.g., 1, 6, 12 months). Collect biomarkers (e.g., inflammatory cytokines, organ histopathology) and employ survival analysis (Kaplan-Meier curves) to evaluate toxicity. Include sham and positive control groups to distinguish this compound-specific effects from background noise .
Q. Methodological Considerations
- Data Management : Develop a Data Management Plan (DMP) specifying storage formats (e.g., .fastq for sequencing), metadata standards (ISA-Tab), and ethical guidelines for sharing .
- Theoretical Frameworks : Anchor studies in established theories (e.g., Lipid Nanoparticle Self-Assembly Theory) to guide hypothesis generation and observational criteria .
- Ethical Compliance : Obtain institutional review board (IRB) approval for human cell line usage and adhere to GDPR for data anonymization .
Properties
IUPAC Name |
2,3-bis[(Z)-octadec-9-enoxy]propyl-trimethylazanium;chloride | |
---|---|---|
Source | PubChem | |
URL | https://pubchem.ncbi.nlm.nih.gov | |
Description | Data deposited in or computed by PubChem | |
InChI |
InChI=1S/C42H84NO2.ClH/c1-6-8-10-12-14-16-18-20-22-24-26-28-30-32-34-36-38-44-41-42(40-43(3,4)5)45-39-37-35-33-31-29-27-25-23-21-19-17-15-13-11-9-7-2;/h20-23,42H,6-19,24-41H2,1-5H3;1H/q+1;/p-1/b22-20-,23-21-; | |
Source | PubChem | |
URL | https://pubchem.ncbi.nlm.nih.gov | |
Description | Data deposited in or computed by PubChem | |
InChI Key |
LDGWQMRUWMSZIU-LQDDAWAPSA-M | |
Source | PubChem | |
URL | https://pubchem.ncbi.nlm.nih.gov | |
Description | Data deposited in or computed by PubChem | |
Canonical SMILES |
CCCCCCCCC=CCCCCCCCCOCC(C[N+](C)(C)C)OCCCCCCCCC=CCCCCCCCC.[Cl-] | |
Source | PubChem | |
URL | https://pubchem.ncbi.nlm.nih.gov | |
Description | Data deposited in or computed by PubChem | |
Isomeric SMILES |
CCCCCCCC/C=C\CCCCCCCCOCC(C[N+](C)(C)C)OCCCCCCCC/C=C\CCCCCCCC.[Cl-] | |
Source | PubChem | |
URL | https://pubchem.ncbi.nlm.nih.gov | |
Description | Data deposited in or computed by PubChem | |
Molecular Formula |
C42H84ClNO2 | |
Source | PubChem | |
URL | https://pubchem.ncbi.nlm.nih.gov | |
Description | Data deposited in or computed by PubChem | |
Molecular Weight |
670.6 g/mol | |
Source | PubChem | |
URL | https://pubchem.ncbi.nlm.nih.gov | |
Description | Data deposited in or computed by PubChem | |
CAS No. |
104162-48-3 | |
Record name | N-(1-(2,3-Dioleyloxy)propyl)-N,N,N-trimethylammonium | |
Source | ChemIDplus | |
URL | https://pubchem.ncbi.nlm.nih.gov/substance/?source=chemidplus&sourceid=0104162483 | |
Description | ChemIDplus is a free, web search system that provides access to the structure and nomenclature authority files used for the identification of chemical substances cited in National Library of Medicine (NLM) databases, including the TOXNET system. | |
Retrosynthesis Analysis
AI-Powered Synthesis Planning: Our tool employs the Template_relevance Pistachio, Template_relevance Bkms_metabolic, Template_relevance Pistachio_ringbreaker, Template_relevance Reaxys, Template_relevance Reaxys_biocatalysis model, leveraging a vast database of chemical reactions to predict feasible synthetic routes.
One-Step Synthesis Focus: Specifically designed for one-step synthesis, it provides concise and direct routes for your target compounds, streamlining the synthesis process.
Accurate Predictions: Utilizing the extensive PISTACHIO, BKMS_METABOLIC, PISTACHIO_RINGBREAKER, REAXYS, REAXYS_BIOCATALYSIS database, our tool offers high-accuracy predictions, reflecting the latest in chemical research and data.
Strategy Settings
Precursor scoring | Relevance Heuristic |
---|---|
Min. plausibility | 0.01 |
Model | Template_relevance |
Template Set | Pistachio/Bkms_metabolic/Pistachio_ringbreaker/Reaxys/Reaxys_biocatalysis |
Top-N result to add to graph | 6 |
Feasible Synthetic Routes
Disclaimer and Information on In-Vitro Research Products
Please be aware that all articles and product information presented on BenchChem are intended solely for informational purposes. The products available for purchase on BenchChem are specifically designed for in-vitro studies, which are conducted outside of living organisms. In-vitro studies, derived from the Latin term "in glass," involve experiments performed in controlled laboratory settings using cells or tissues. It is important to note that these products are not categorized as medicines or drugs, and they have not received approval from the FDA for the prevention, treatment, or cure of any medical condition, ailment, or disease. We must emphasize that any form of bodily introduction of these products into humans or animals is strictly prohibited by law. It is essential to adhere to these guidelines to ensure compliance with legal and ethical standards in research and experimentation.