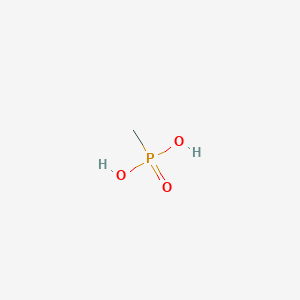
Methylphosphonic acid
Overview
Description
Methylphosphonic acid is an organophosphorus compound with the chemical formula CH₃P(O)(OH)₂. It is characterized by a tetrahedral phosphorus center bonded to a methyl group, two hydroxyl groups, and an oxygen atom. This compound is a white, non-volatile solid that is poorly soluble in organic solvents but soluble in water and common alcohols .
Mechanism of Action
Target of Action
Methylphosphonic acid (MPn) is an organophosphorus compound with the chemical formula CH3P(O)(OH)2 . The primary targets of this compound are Cholinesterase and Acetylcholinesterase in humans, and Esterase in Streptomyces scabies .
Mode of Action
It’s known that the phosphorus center of the compound is tetrahedral and is bonded to a methyl group, two oh groups, and an oxygen . This structure allows it to interact with its targets in a unique way.
Biochemical Pathways
This compound is involved in the global phosphorus cycle and in oceanic methane production . It is synthesized by marine microbes and is an intermediate in the synthesis of phosphorus-containing nerve agents, such as sarin and VX . The rich biochemistry that has evolved to synthesize and catabolize this compound into Pi and methane involves reactions catalyzed by This compound synthase MpnS and the carbon–phosphorus lyase system .
Pharmacokinetics
It is known that this compound is a white, non-volatile solid that is poorly soluble in organic solvents but soluble in water and common alcohols . This suggests that its bioavailability may be influenced by these properties.
Result of Action
The result of this compound’s action is the cleavage of the C-P bond, leading to the production of Pi and methane . This process is significant in the context of global climate change and eutrophication .
Action Environment
The action of this compound is influenced by environmental factors. For instance, the extent of MPn degradation was found to be more under alkaline conditions . Additionally, exposure to moist air or water can also influence the action of this compound .
Biochemical Analysis
Biochemical Properties
Methylphosphonic acid is very stable . It plays an important role in aquatic systems like rivers or the open ocean . The vibrational spectra of aqueous solutions of this compound and its anions have been studied .
Cellular Effects
It is known that the microbial metabolism of this compound has a significant link to the methane production in the open ocean and the large-scale biogeochemical cycles of key elements .
Molecular Mechanism
The molecular mechanism of this compound involves the cleavage of the C–P bond under UV irradiation . Reactive oxygen species (ROS) are involved in the photo-oxidation reaction process .
Temporal Effects in Laboratory Settings
The degradation kinetics results indicated that the extent of this compound degradation was more under alkaline conditions and that the degradation process was more rapid at the initial stage of the reaction .
Metabolic Pathways
This compound is involved in the phosphorus cycle . It is a precursor to more complex phosphonate molecules, such as glyphosate .
Preparation Methods
Synthetic Routes and Reaction Conditions: Methylphosphonic acid can be synthesized from triethylphosphite through a Michaelis-Arbuzov reaction, which generates the phosphorus (V) center: [ \text{CH}_3\text{Cl} + \text{P(OC}_2\text{H}_5)_3 \rightarrow \text{CH}_3\text{PO(OC}_2\text{H}_5)_2 + \text{C}_2\text{H}_5\text{Cl} ] The resulting dialkylphosphonate is then treated with chlorotrimethylsilane before hydrolysis of the siloxyphosphonate to generate the desired product: [ \text{CH}_3\text{PO(OC}_2\text{H}_5)_2 + 2 \text{Me}_3\text{SiCl} \rightarrow \text{CH}_3\text{PO(OSiMe}_3)_2 + 2 \text{C}_2\text{H}_5\text{Cl} ] [ \text{CH}_3\text{PO(OSiMe}_3)_2 + 2 \text{H}_2\text{O} \rightarrow \text{CH}_3\text{PO(OH)}_2 + 2 \text{HOSiMe}_3 ] This reaction pathway proceeds via the siloxyphosphonate intermediate due to the difficulty in directly hydrolyzing dialkylphosphonates .
Industrial Production Methods: Industrial production of this compound often involves the dealkylation of dialkyl phosphonates under acidic conditions (using hydrochloric acid) or through the McKenna procedure, which involves a two-step reaction using bromotrimethylsilane followed by methanolysis .
Chemical Reactions Analysis
Types of Reactions: Methylphosphonic acid undergoes various chemical reactions, including:
Oxidation: It can be oxidized to form phosphoric acid derivatives.
Reduction: It can be reduced to form phosphine derivatives.
Substitution: It can undergo nucleophilic substitution reactions where the hydroxyl groups are replaced by other nucleophiles.
Common Reagents and Conditions:
Oxidation: Common oxidizing agents include hydrogen peroxide and potassium permanganate.
Reduction: Reducing agents such as lithium aluminum hydride can be used.
Substitution: Reagents like alkyl halides and acyl chlorides are commonly used in substitution reactions.
Major Products:
Oxidation: Phosphoric acid derivatives.
Reduction: Phosphine derivatives.
Substitution: Various substituted phosphonic acids
Scientific Research Applications
Methylphosphonic acid has a wide range of scientific research applications:
Chemistry: It is used as an intermediate in the synthesis of more complex phosphonate molecules, such as glyphosate.
Biology: It is a biosynthesis product of marine microorganisms and plays a role in the biogeochemical cycles of key elements like iron, nitrogen, and phosphorus.
Medicine: It is an intermediate in the synthesis of phosphorus-containing nerve agents, such as sarin and VX.
Industry: It is used in the production of flame retardants, plasticizers, and corrosion inhibitors
Comparison with Similar Compounds
- Ethylphosphonic acid
- Dimethylphosphonic acid
- Aminothis compound
Comparison: this compound is unique due to its simple structure and stability. It serves as a precursor to more complex phosphonate molecules and has significant applications in various fields. Compared to ethylphosphonic acid and dithis compound, this compound is more stable and less volatile. Aminothis compound, on the other hand, has additional functional groups that make it more reactive and suitable for different applications .
Biological Activity
Methylphosphonic acid (MPn) is a compound of significant interest due to its biological activity, particularly in marine ecosystems and its implications in environmental chemistry. This article examines the biological mechanisms, microbial interactions, and potential environmental impacts associated with MPn, supported by relevant data and case studies.
Overview of this compound
This compound is primarily known as an intermediate in the synthesis of nerve agents such as sarin and VX. However, it also plays a crucial role in marine biochemistry, particularly concerning phosphorus cycling and methane production. Its unique structure allows it to participate in various biochemical pathways, particularly those involving microbial metabolism.
Microbial Catabolism
Recent studies have highlighted the ability of certain marine microbes to utilize this compound as a phosphorus source. For instance, the archaeon Nitrosopumilus maritimus has been shown to encode pathways for methylphosphonate biosynthesis, indicating that this compound is more prevalent in marine environments than previously understood .
Key Findings:
- Methane Production: The catabolism of MPn by phosphorus-starved marine microbes leads to methane production, which is significant given the greenhouse gas implications. This phenomenon has been termed the "methane paradox" because it suggests that MPn could be a substantial source of methane in oxic marine waters .
- Enzymatic Pathways: The enzyme carbon-phosphorus (C-P) lyase facilitates the breakdown of this compound, releasing methane as a byproduct. This pathway is supported by the presence of C-P lyase genes in many marine microbial communities .
Photo-degradation and Reactive Oxygen Species
This compound undergoes photo-degradation under UV irradiation, which can lead to its transformation into reactive intermediates that may have further ecological consequences. A study utilizing multi-labeled water isotope probing demonstrated that reactive oxygen species (ROS) play a critical role in the cleavage of the C–P bond in MPn .
Data Table: Reaction Rates Under Different Conditions
Condition | Inhibition Rate (%) | Rate Constant (h⁻¹) |
---|---|---|
Blank (pH 3) | — | 0.98 × 10⁻² |
Isopropanol (pH 3) | 92.86 | 0.07 × 10⁻² |
NaN₃ (pH 3) | 68.37 | 0.31 × 10⁻² |
Blank (pH 8) | — | 1.77 × 10⁻² |
Isopropanol (pH 8) | 96.61 | 0.06 × 10⁻² |
NaN₃ (pH 8) | 66.1 | 0.6 × 10⁻² |
This table illustrates how different scavengers affect the degradation rate of MPn under varying pH conditions, indicating that alkaline conditions favor C–P bond cleavage more than acidic conditions.
Marine Ecosystem Dynamics
A study conducted across various ocean depths revealed that methylphosphonate utilization significantly contributes to methane formation rates in marine environments . The research showed immediate and linear increases in methane production upon incubation with MPn, highlighting its role in nutrient cycling.
Experimental Design:
- Incubation Conditions: Samples were taken from different ocean depths to assess methane production rates.
- Results: Methane formation was observed to be consistent across samples, suggesting widespread microbial utilization of MPn.
Properties
IUPAC Name |
methylphosphonic acid | |
---|---|---|
Source | PubChem | |
URL | https://pubchem.ncbi.nlm.nih.gov | |
Description | Data deposited in or computed by PubChem | |
InChI |
InChI=1S/CH5O3P/c1-5(2,3)4/h1H3,(H2,2,3,4) | |
Source | PubChem | |
URL | https://pubchem.ncbi.nlm.nih.gov | |
Description | Data deposited in or computed by PubChem | |
InChI Key |
YACKEPLHDIMKIO-UHFFFAOYSA-N | |
Source | PubChem | |
URL | https://pubchem.ncbi.nlm.nih.gov | |
Description | Data deposited in or computed by PubChem | |
Canonical SMILES |
CP(=O)(O)O | |
Source | PubChem | |
URL | https://pubchem.ncbi.nlm.nih.gov | |
Description | Data deposited in or computed by PubChem | |
Molecular Formula |
CH5O3P | |
Source | PubChem | |
URL | https://pubchem.ncbi.nlm.nih.gov | |
Description | Data deposited in or computed by PubChem | |
DSSTOX Substance ID |
DTXSID5047748 | |
Record name | Methyl phosphonic acid | |
Source | EPA DSSTox | |
URL | https://comptox.epa.gov/dashboard/DTXSID5047748 | |
Description | DSSTox provides a high quality public chemistry resource for supporting improved predictive toxicology. | |
Molecular Weight |
96.022 g/mol | |
Source | PubChem | |
URL | https://pubchem.ncbi.nlm.nih.gov | |
Description | Data deposited in or computed by PubChem | |
Physical Description |
Liquid, White solid; [HSDB] White flakes or crystalline solid; [MSDSonline] | |
Record name | Phosphonic acid, P-methyl- | |
Source | EPA Chemicals under the TSCA | |
URL | https://www.epa.gov/chemicals-under-tsca | |
Description | EPA Chemicals under the Toxic Substances Control Act (TSCA) collection contains information on chemicals and their regulations under TSCA, including non-confidential content from the TSCA Chemical Substance Inventory and Chemical Data Reporting. | |
Record name | Methylphosphonic acid | |
Source | Haz-Map, Information on Hazardous Chemicals and Occupational Diseases | |
URL | https://haz-map.com/Agents/6137 | |
Description | Haz-Map® is an occupational health database designed for health and safety professionals and for consumers seeking information about the adverse effects of workplace exposures to chemical and biological agents. | |
Explanation | Copyright (c) 2022 Haz-Map(R). All rights reserved. Unless otherwise indicated, all materials from Haz-Map are copyrighted by Haz-Map(R). No part of these materials, either text or image may be used for any purpose other than for personal use. Therefore, reproduction, modification, storage in a retrieval system or retransmission, in any form or by any means, electronic, mechanical or otherwise, for reasons other than personal use, is strictly prohibited without prior written permission. | |
Boiling Point |
Decomposes | |
Record name | METHYL PHOSPHONIC ACID | |
Source | Hazardous Substances Data Bank (HSDB) | |
URL | https://pubchem.ncbi.nlm.nih.gov/source/hsdb/6762 | |
Description | The Hazardous Substances Data Bank (HSDB) is a toxicology database that focuses on the toxicology of potentially hazardous chemicals. It provides information on human exposure, industrial hygiene, emergency handling procedures, environmental fate, regulatory requirements, nanomaterials, and related areas. The information in HSDB has been assessed by a Scientific Review Panel. | |
Solubility |
Soluble in ethanol and ethyl ether; insoluble in benzene, In water, >20,000 mg/l (no temperature reported) | |
Record name | METHYL PHOSPHONIC ACID | |
Source | Hazardous Substances Data Bank (HSDB) | |
URL | https://pubchem.ncbi.nlm.nih.gov/source/hsdb/6762 | |
Description | The Hazardous Substances Data Bank (HSDB) is a toxicology database that focuses on the toxicology of potentially hazardous chemicals. It provides information on human exposure, industrial hygiene, emergency handling procedures, environmental fate, regulatory requirements, nanomaterials, and related areas. The information in HSDB has been assessed by a Scientific Review Panel. | |
Vapor Pressure |
0.000002 [mmHg] | |
Record name | Methylphosphonic acid | |
Source | Haz-Map, Information on Hazardous Chemicals and Occupational Diseases | |
URL | https://haz-map.com/Agents/6137 | |
Description | Haz-Map® is an occupational health database designed for health and safety professionals and for consumers seeking information about the adverse effects of workplace exposures to chemical and biological agents. | |
Explanation | Copyright (c) 2022 Haz-Map(R). All rights reserved. Unless otherwise indicated, all materials from Haz-Map are copyrighted by Haz-Map(R). No part of these materials, either text or image may be used for any purpose other than for personal use. Therefore, reproduction, modification, storage in a retrieval system or retransmission, in any form or by any means, electronic, mechanical or otherwise, for reasons other than personal use, is strictly prohibited without prior written permission. | |
Color/Form |
White solid | |
CAS No. |
993-13-5 | |
Record name | Methylphosphonic acid | |
Source | CAS Common Chemistry | |
URL | https://commonchemistry.cas.org/detail?cas_rn=993-13-5 | |
Description | CAS Common Chemistry is an open community resource for accessing chemical information. Nearly 500,000 chemical substances from CAS REGISTRY cover areas of community interest, including common and frequently regulated chemicals, and those relevant to high school and undergraduate chemistry classes. This chemical information, curated by our expert scientists, is provided in alignment with our mission as a division of the American Chemical Society. | |
Explanation | The data from CAS Common Chemistry is provided under a CC-BY-NC 4.0 license, unless otherwise stated. | |
Record name | Methyl phosphonic acid | |
Source | ChemIDplus | |
URL | https://pubchem.ncbi.nlm.nih.gov/substance/?source=chemidplus&sourceid=0000993135 | |
Description | ChemIDplus is a free, web search system that provides access to the structure and nomenclature authority files used for the identification of chemical substances cited in National Library of Medicine (NLM) databases, including the TOXNET system. | |
Record name | Methylphosphonic acid | |
Source | DTP/NCI | |
URL | https://dtp.cancer.gov/dtpstandard/servlet/dwindex?searchtype=NSC&outputformat=html&searchlist=119358 | |
Description | The NCI Development Therapeutics Program (DTP) provides services and resources to the academic and private-sector research communities worldwide to facilitate the discovery and development of new cancer therapeutic agents. | |
Explanation | Unless otherwise indicated, all text within NCI products is free of copyright and may be reused without our permission. Credit the National Cancer Institute as the source. | |
Record name | Phosphonic acid, P-methyl- | |
Source | EPA Chemicals under the TSCA | |
URL | https://www.epa.gov/chemicals-under-tsca | |
Description | EPA Chemicals under the Toxic Substances Control Act (TSCA) collection contains information on chemicals and their regulations under TSCA, including non-confidential content from the TSCA Chemical Substance Inventory and Chemical Data Reporting. | |
Record name | Methyl phosphonic acid | |
Source | EPA DSSTox | |
URL | https://comptox.epa.gov/dashboard/DTXSID5047748 | |
Description | DSSTox provides a high quality public chemistry resource for supporting improved predictive toxicology. | |
Record name | Methylphosphonic acid | |
Source | European Chemicals Agency (ECHA) | |
URL | https://echa.europa.eu/substance-information/-/substanceinfo/100.012.370 | |
Description | The European Chemicals Agency (ECHA) is an agency of the European Union which is the driving force among regulatory authorities in implementing the EU's groundbreaking chemicals legislation for the benefit of human health and the environment as well as for innovation and competitiveness. | |
Explanation | Use of the information, documents and data from the ECHA website is subject to the terms and conditions of this Legal Notice, and subject to other binding limitations provided for under applicable law, the information, documents and data made available on the ECHA website may be reproduced, distributed and/or used, totally or in part, for non-commercial purposes provided that ECHA is acknowledged as the source: "Source: European Chemicals Agency, http://echa.europa.eu/". Such acknowledgement must be included in each copy of the material. ECHA permits and encourages organisations and individuals to create links to the ECHA website under the following cumulative conditions: Links can only be made to webpages that provide a link to the Legal Notice page. | |
Record name | METHYLPHOSPHONIC ACID | |
Source | FDA Global Substance Registration System (GSRS) | |
URL | https://gsrs.ncats.nih.gov/ginas/app/beta/substances/329W4YM10Z | |
Description | The FDA Global Substance Registration System (GSRS) enables the efficient and accurate exchange of information on what substances are in regulated products. Instead of relying on names, which vary across regulatory domains, countries, and regions, the GSRS knowledge base makes it possible for substances to be defined by standardized, scientific descriptions. | |
Explanation | Unless otherwise noted, the contents of the FDA website (www.fda.gov), both text and graphics, are not copyrighted. They are in the public domain and may be republished, reprinted and otherwise used freely by anyone without the need to obtain permission from FDA. Credit to the U.S. Food and Drug Administration as the source is appreciated but not required. | |
Record name | METHYL PHOSPHONIC ACID | |
Source | Hazardous Substances Data Bank (HSDB) | |
URL | https://pubchem.ncbi.nlm.nih.gov/source/hsdb/6762 | |
Description | The Hazardous Substances Data Bank (HSDB) is a toxicology database that focuses on the toxicology of potentially hazardous chemicals. It provides information on human exposure, industrial hygiene, emergency handling procedures, environmental fate, regulatory requirements, nanomaterials, and related areas. The information in HSDB has been assessed by a Scientific Review Panel. | |
Melting Point |
108.5 °C | |
Record name | METHYL PHOSPHONIC ACID | |
Source | Hazardous Substances Data Bank (HSDB) | |
URL | https://pubchem.ncbi.nlm.nih.gov/source/hsdb/6762 | |
Description | The Hazardous Substances Data Bank (HSDB) is a toxicology database that focuses on the toxicology of potentially hazardous chemicals. It provides information on human exposure, industrial hygiene, emergency handling procedures, environmental fate, regulatory requirements, nanomaterials, and related areas. The information in HSDB has been assessed by a Scientific Review Panel. | |
Retrosynthesis Analysis
AI-Powered Synthesis Planning: Our tool employs the Template_relevance Pistachio, Template_relevance Bkms_metabolic, Template_relevance Pistachio_ringbreaker, Template_relevance Reaxys, Template_relevance Reaxys_biocatalysis model, leveraging a vast database of chemical reactions to predict feasible synthetic routes.
One-Step Synthesis Focus: Specifically designed for one-step synthesis, it provides concise and direct routes for your target compounds, streamlining the synthesis process.
Accurate Predictions: Utilizing the extensive PISTACHIO, BKMS_METABOLIC, PISTACHIO_RINGBREAKER, REAXYS, REAXYS_BIOCATALYSIS database, our tool offers high-accuracy predictions, reflecting the latest in chemical research and data.
Strategy Settings
Precursor scoring | Relevance Heuristic |
---|---|
Min. plausibility | 0.01 |
Model | Template_relevance |
Template Set | Pistachio/Bkms_metabolic/Pistachio_ringbreaker/Reaxys/Reaxys_biocatalysis |
Top-N result to add to graph | 6 |
Feasible Synthetic Routes
Q1: What is the primary environmental concern regarding methylphosphonic acid (MPA)?
A1: this compound (MPA) is a significant environmental concern due to its presence as a degradation product of organophosphorus nerve agents like Sarin (GB) and VX. [, , , , , ] Detecting MPA in the environment can indicate the past use or production of these dangerous chemical warfare agents. [, ]
Q2: Are there alternative derivatization techniques for analyzing MPA?
A3: Researchers are exploring faster and more efficient derivatization techniques for MPA. One promising approach involves converting MPA into its dialkyl esters using thionyl chloride followed by reaction with various alcohols like methanol, ethanol, and butanol. [] This method offers a potentially less hazardous and more cost-effective alternative to traditional techniques. []
Q3: Can liquid chromatography be used to analyze MPA and related compounds?
A4: Yes, reversed-phase ion-pairing high-performance liquid chromatography (RP-IP-HPLC), coupled with inductively coupled plasma mass spectrometry (ICP-MS), has been successfully used to separate and analyze MPA, ethyl this compound (EMPA), and isopropyl this compound (IMPA). [] This method utilizes a myristyl trimethylammonium bromide ion-pairing reagent and an ammonium acetate-acetic acid buffer system. []
Q4: How do different alkyl methylphosphonic acids differ in their detection limits using RP-IP-HPLC-ICP-MS?
A6: Using the RP-IP-HPLC-ICP-MS method, detection limits vary for different alkyl MPAs. For instance, the detection limit for EMPA is 263 pg/mL, for IMPA it is 183 pg/mL, and for MPA it is 139 pg/mL. []
Q5: What is the significance of this compound in biological systems?
A7: this compound is not naturally found in biological systems and is considered biologically inert. [] Studies have shown that rats do not degrade MPA into phosphoric acid, and it is excreted unchanged in their urine. []
Q6: How does the carbon-phosphorus lyase pathway contribute to the utilization of this compound?
A8: Some marine bacteria utilize MPA as a source of inorganic phosphate (Pi) through the carbon-phosphorus lyase pathway. [] This pathway cleaves the carbon-phosphorus bond, releasing methane as a byproduct and generating Pi for the bacteria. []
Q7: Is there an alternative pathway for the catabolism of this compound?
A9: Recent research has unveiled an oxidative pathway for MPA catabolism in the bacterium Gimesia maris. [] This pathway, facilitated by non-heme Fe(II)-dependent oxygenases GmPhnY* and GmPhnZ1, oxidizes MPA to hydroxythis compound and then to formic acid and Pi. []
Q8: How do the substrate specificities of GmPhnY* and GmPhnZ1 contribute to the understanding of this compound metabolism?
A10: GmPhnY* specifically acts on MPA, converting it to hydroxythis compound. [] GmPhnZ1 then oxidizes hydroxythis compound, ultimately yielding formic acid and Pi. [] Notably, 2-aminoethylphosphonic acid is not a substrate for this pathway. []
Q9: What role does 31P NMR spectroscopy play in the study of this compound metabolism?
A11: 31P NMR spectroscopy is an essential tool for studying MPA metabolism. [] It allows researchers to monitor the transformation of MPA and its intermediates, such as hydroxythis compound, during the oxidative pathway. []
Q10: How do phosphonic acids interact with calcite crystals?
A12: Molecular dynamics simulations have been employed to investigate the interaction of various phosphonic acids, including nitrilotris(this compound) (NTMP), with calcite crystals. [] These simulations reveal that the binding energy of these scale inhibitors is higher on the (110) face of calcite compared to the (104) face. []
Q11: How does the binding of phosphonic acids to calcite crystals impact scale inhibition?
A13: The preferential binding of phosphonic acids, like NTMP, to specific crystal faces of calcite (e.g., (110)) suggests a mechanism for their scale inhibition properties. [] By binding to these faces, they can disrupt crystal growth and prevent the formation of scale deposits. []
Q12: What insights do pair correlation function analyses provide regarding phosphonic acid-calcite interactions?
A14: Analyses of pair correlation functions from molecular dynamics simulations support the observed binding energy differences of phosphonic acids on different calcite crystal faces. [] This provides further evidence for the preferential binding of these inhibitors to specific crystallographic planes. []
Q13: What is the relative scale inhibition efficiency of various phosphonic acids against calcite?
A15: Molecular dynamics simulations suggest the following order of scale inhibition efficiency against calcite for various phosphonic acids: 1,2-ethylenediamine-N,N,N′,N′-tetrakis(this compound) (EDATMP) > 1,6-hexylenediamine-N,N,N′,N′-tetrakis- (this compound) (HDATMP) > 1- hydroxy-1,1-ethylenebis(phosphonic acid) (HEBP) > NTMP > N,N-glycine-bis(this compound) (GBMP) > 1-amino-1,1-ethylenebis(phosphonic acid) (AEBP) > nitrilo-methyl-bis(this compound) (NMBMP). []
Q14: How do phosphonic acids structurally change upon binding to calcite surfaces?
A16: Molecular dynamics simulations indicate that phosphonic acid molecules undergo conformational changes during the binding process to calcite crystal surfaces. [] This highlights the dynamic nature of these interactions and their importance for effective scale inhibition. []
Q15: What type of chemical bonds are involved in the interaction between phosphonic acids and calcite crystals?
A17: The interaction between phosphonic acids and calcite crystals involves the formation of electrovalent bonds. [] These bonds occur between the phosphoryl oxygen atoms in the phosphonic acid molecules and the calcium ions present on the calcite crystal surface. []
Q16: How do the properties of this compound affect its analytical detection?
A18: Due to its high polarity, MPA poses challenges for direct analysis using techniques like gas chromatography. [, , ] To overcome this, derivatization methods, such as esterification or silylation, are commonly employed to convert MPA into less polar, more volatile derivatives suitable for GC-MS analysis. [, , , ]
Q17: What is the role of trimethylsilyldiazomethane in the analysis of alkyl methylphosphonic acids?
A19: Trimethylsilyldiazomethane is a useful reagent for derivatizing alkyl methylphosphonic acids, converting them into their corresponding methyl esters. [] These methyl ester derivatives are more amenable to analysis by gas chromatography-mass spectrometry (GC-MS), facilitating the identification and quantification of these compounds. []
Q18: Can matrix-assisted laser desorption ionization time-of-flight mass spectrometry (MALDI-TOFMS) be used for the analysis of nerve agent degradation products?
A20: Yes, MALDI-TOFMS has shown promise as a rapid screening method for detecting nerve agent degradation products, including alkyl methylphosphonic acids. [, ] Using matrices like α-cyano-4-hydroxycinnamic acid (CHCA) or 7-(diethylamino)coumarin-3-carboxylic acid (DCCA) enables the ionization and detection of these compounds. [, ]
Q19: How do different laser systems influence the detection of nerve agent degradation products in MALDI-TOFMS?
A21: The choice of laser system in MALDI-TOFMS can impact the detection of nerve agent degradation products. For instance, the third harmonic generation (355 nm) of a Nd:YAG laser is often used in conjunction with the CHCA matrix. [] Conversely, a hydrogen Raman laser (multifrequency laser) might be employed with the DCCA matrix for optimal ionization and detection. []
Q20: What advantages does paper spray mass spectrometry (PS-MS) offer for analyzing chemical warfare agents and their hydrolysis products?
A22: PS-MS presents several advantages for analyzing chemical warfare agents and their hydrolysis products in biological samples like blood and urine. [] It requires minimal sample preparation, offers rapid analysis, and can detect these compounds at physiologically relevant concentrations. []
Q21: What are the benefits of using isotopically labeled internal standards in the analysis of chemical warfare agents and their degradation products?
A23: Isotopically labeled internal standards play a crucial role in ensuring accurate and reliable quantification of chemical warfare agents and their degradation products. [, ] These standards, added to the analyzed sample, allow for correction of variations during sample preparation and analysis, leading to more precise and reliable results. [, ]
Q22: How does the addition of chlorinated solvents affect the performance of paper spray mass spectrometry (PS-MS) in the negative ion mode?
A24: Incorporating chlorinated solvents in the PS-MS analysis, particularly in the negative ion mode, can enhance the stability and robustness of the technique. [] This improvement facilitates the detection of chemical warfare agent hydrolysis products at low concentrations in complex matrices like blood and urine. []
Q23: How does the structure of (o-hydroxyphenyl)methylphosphonic acids influence their deprotonation sequence?
A25: The deprotonation sequence of (o-hydroxyphenyl)methylphosphonic acids is governed by intramolecular hydrogen bonding involving the phenolic OH group. [] Depending on the position and nature of substituents on the phenyl ring, the deprotonation can occur from either the phenolic OH or the POH group, influencing the overall charge and reactivity of the molecule. []
Q24: What spectroscopic techniques are used to study the deprotonation of (o-hydroxyphenyl)methylphosphonic acids?
A26: UV/VIS absorption spectroscopy is a valuable tool for studying the deprotonation of (o-hydroxyphenyl)methylphosphonic acids. [] By monitoring changes in the absorption spectra as a function of pH, researchers can determine the dissociation constants (pKa values) and identify the different protonation states of these compounds. []
Q25: What techniques are employed to characterize the complexation between cerium(III) ions and aminophosphonic acids?
A29: Luminescence spectroscopy is a valuable technique for characterizing the complexation between Ce3+ and aminophosphonic acids. [] By monitoring changes in the emission intensity of Ce3+ upon titrating with the ligand, researchers can determine the stoichiometry and stability of the formed complexes. []
Q26: What in vitro assays are employed to assess the efficacy of aminophosphonic acids as anti-insect agents?
A31: The efficacy of aminophosphonic acids as anti-insect agents can be assessed using in vitro assays that measure their ability to inhibit the activity of insect aminopeptidases. [] By monitoring the enzyme's activity in the presence of varying concentrations of the aminophosphonic acid, researchers can determine the compound's inhibitory potency (e.g., IC50 values). []
Q27: How does the protection of cyclam with the phosphonothioyl group facilitate the synthesis of 1,4-H4te2p?
A33: The phosphonothioyl group serves as a protecting group for cyclam during the synthesis of 1,4-H4te2p. [] This protection strategy allows for selective functionalization of the cyclam ring, leading to the desired bis(this compound) derivative with high regioselectivity. []
Q28: What insights do crystal structure analyses provide regarding the conformation and hydrogen bonding patterns in cyclam-based phosphonic acid derivatives?
A34: Crystal structure analyses of various cyclam-based phosphonic acid derivatives, including 1,4-H4te2p and 1,4,8,11-tetraazacyclotetradecane-1,4,8,11-tetrakis(this compound) (H8tetp), reveal a consistent ring conformation and specific hydrogen bonding interactions. [] These interactions, often between phosphonate oxygen atoms and protonated nitrogen atoms within the cyclam ring, influence the stability and solution properties of these compounds. []
Q29: What is the significance of selective inhibition of autotaxin by these this compound derivatives?
A36: The selective inhibition of ATX by benzyl and naphthalene this compound derivatives is crucial for targeted therapeutic intervention. [] By selectively targeting ATX, these compounds can potentially reduce LPA-mediated tumor cell invasion and metastasis without affecting the activity of related enzymes, thereby minimizing off-target effects. []
Q30: What are the potential therapeutic implications of inhibiting autotaxin in cancer?
A37: Inhibiting ATX, an enzyme overexpressed in various cancers, represents a promising therapeutic strategy for managing cancer progression. [] By reducing LPA production, ATX inhibitors can potentially limit tumor cell growth, invasion, and metastasis, leading to improved treatment outcomes for cancer patients. []
Q31: What in vitro and in vivo models are used to evaluate the efficacy of autotaxin inhibitors?
A38: The efficacy of ATX inhibitors, such as benzyl and naphthalene this compound derivatives, is evaluated using various in vitro and in vivo models. [] In vitro assays may involve measuring the ability of these compounds to inhibit ATX enzymatic activity or to block the invasion of cancer cells. [] In vivo studies, often conducted in mouse models, can assess the impact of these inhibitors on tumor growth and metastasis. []
Q32: How do molecular probes containing deuterium-labeled this compound contribute to understanding the mechanism of action of chemical threat agents?
A39: Molecular probes incorporating deuterium-labeled this compound, such as (methyl-d3)ethyl this compound and (methyl-d3)pinacolyl this compound, are valuable tools for elucidating the mechanism of action of chemical threat agents. [] By introducing these labeled compounds into biological systems, researchers can track their metabolic fate and identify specific interactions with enzymes or target molecules. []
Q33: How do Fourier transform infrared (FT-IR) and Raman spectroscopies contribute to understanding the structure and bonding in this compound derivatives?
A41: FT-IR and Raman spectroscopies are complementary techniques that provide valuable information about the vibrational modes of molecules, revealing insights into their structure and bonding. [] When applied to this compound derivatives, these techniques can help identify characteristic functional groups, such as P-O, P=O, and C-H vibrations, and provide information about hydrogen bonding interactions. []
Q34: What structural insights can be gained from comparing the solid-state and solution-phase spectroscopic data of this compound derivatives?
A42: Comparing spectroscopic data obtained in the solid state and solution phase can provide valuable insights into the intermolecular interactions and conformational changes that occur upon solvation. [, ] For instance, shifts in vibrational frequencies or changes in band intensities can indicate the formation or disruption of hydrogen bonds when this compound derivatives are dissolved in various solvents. [, ]
Q35: How does the presence of a boron atom in the aromatic ring of aminophosphonic acids influence their spectroscopic properties and adsorption behavior?
A43: The incorporation of a boron atom into the aromatic ring of aminophosphonic acids can significantly impact their spectroscopic properties and adsorption behavior. [] The presence of boron can alter the electronic distribution within the molecule, influencing its vibrational frequencies and interactions with metal surfaces. [] These changes can be probed using techniques like FT-IR, Raman, and SERS to understand the influence of boron on the properties and potential applications of these modified aminophosphonic acids. []
Q36: How does the absolute configuration of chiral this compound derivatives affect their interaction with metal surfaces?
A44: The absolute configuration of chiral this compound derivatives can significantly influence their adsorption geometry and interaction strength with metal surfaces. [] This difference in adsorption behavior can arise from steric effects, differences in electronic properties, or specific interactions between the chiral center and the metal surface. [] Understanding these chiral effects is crucial for designing and optimizing these compounds for applications where surface interactions play a critical role, such as in catalysis or sensing. []
Q37: How can the concentration and pH of the solution influence the surface-enhanced Raman scattering (SERS) spectra of this compound derivatives?
A45: The SERS spectra of this compound derivatives can be sensitive to changes in the concentration and pH of the surrounding solution. [] Variations in concentration can affect the surface coverage and orientation of the molecules on the metal substrate, leading to changes in SERS intensities and band positions. [] Changes in pH can alter the protonation state of the molecules, influencing their charge distribution, interaction with the metal surface, and consequently, their SERS spectra. []
Q38: How do the spectroscopic features of this compound derivatives adsorbed on metal surfaces differ from those in their free form?
A46: The spectroscopic features of this compound derivatives can change significantly upon adsorption onto metal surfaces compared to their free form in solution or solid state. [, ] These changes can manifest as shifts in vibrational frequencies, changes in band intensities, or the appearance of new bands. [, ] Such spectral variations provide valuable insights into the nature of the interaction between the molecules and the metal surface, such as the formation of specific chemical bonds or changes in molecular orientation. [, ]
Q39: What is the role of zirconium metal-organic frameworks (MOFs) in the context of nerve agent decomposition?
A47: Zirconium MOFs, particularly UiO-66-NH2, exhibit promising activity in decomposing nerve agents under solid-state conditions. [] The MOF acts as a reactive sorbent, effectively hydrolyzing nerve agents like GD, GA, and VX into less toxic products. []
Q40: How do researchers monitor the decomposition of nerve agents by zirconium MOFs?
A48: Researchers employ various analytical techniques to monitor the decomposition of nerve agents by zirconium MOFs. Phosphorus-31 solid state-magic angle spinning nuclear magnetic resonance (31P SS-MAS NMR) provides structural information about the degradation products. [] In addition, gas chromatography-mass spectrometry (GC-MS) identifies and quantifies the volatile breakdown products formed during the decomposition process. []
Q41: How does the pore size of UiO-66-NH2 influence its ability to decompose nerve agents?
A49: The pore size of UiO-66-NH2 plays a critical role in its ability to decompose nerve agents. [] Its hierarchically microporous structure, with pore sizes ranging from 7 Å to 15 Å, allows for the adsorption and subsequent hydrolysis of nerve agents within its pores. [] This confinement within the MOF's pores facilitates the interaction between the nerve agent molecules and the reactive sites of the MOF, leading to their efficient decomposition. []
Disclaimer and Information on In-Vitro Research Products
Please be aware that all articles and product information presented on BenchChem are intended solely for informational purposes. The products available for purchase on BenchChem are specifically designed for in-vitro studies, which are conducted outside of living organisms. In-vitro studies, derived from the Latin term "in glass," involve experiments performed in controlled laboratory settings using cells or tissues. It is important to note that these products are not categorized as medicines or drugs, and they have not received approval from the FDA for the prevention, treatment, or cure of any medical condition, ailment, or disease. We must emphasize that any form of bodily introduction of these products into humans or animals is strictly prohibited by law. It is essential to adhere to these guidelines to ensure compliance with legal and ethical standards in research and experimentation.