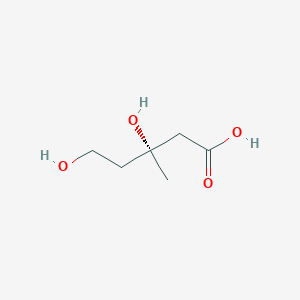
Mevalonic acid
Overview
Description
Preparation Methods
Synthetic Routes and Reaction Conditions: Mevalonic acid can be synthesized through various methods. One common approach involves the enantioselective synthesis starting from isoprenol. The process includes homoallylic epoxidation followed by enantiomeric enrichment to obtain the key epoxide intermediate. Further reactions lead to the production of either racemic this compound or its enantiomers .
Industrial Production Methods: In industrial settings, this compound is often produced via microbial fermentation. This method utilizes genetically engineered microorganisms, such as Escherichia coli, to overexpress the mevalonate pathway enzymes. The fermentation process is optimized to achieve high yields of this compound .
Chemical Reactions Analysis
Types of Reactions: Mevalonic acid undergoes various chemical reactions, including:
Oxidation: this compound can be oxidized to produce mevalonolactone.
Reduction: It can be reduced to form mevalonate.
Phosphorylation: this compound is phosphorylated by mevalonate kinase to form mevalonate-5-phosphate.
Common Reagents and Conditions:
Oxidation: Common oxidizing agents include potassium permanganate and chromium trioxide.
Reduction: Reducing agents such as sodium borohydride are used.
Phosphorylation: ATP is used as a phosphorylating agent in the presence of mevalonate kinase.
Major Products:
Mevalonolactone: Formed through oxidation.
Mevalonate-5-phosphate: Formed through phosphorylation.
Scientific Research Applications
Mevalonic acid has numerous applications in scientific research:
Chemistry: It is used as a precursor in the synthesis of isoprenoids and other complex molecules.
Biology: this compound is essential for the biosynthesis of cholesterol and steroid hormones.
Medicine: It is a target for drugs like statins, which inhibit its production to lower cholesterol levels.
Industry: this compound is used in the production of synthetic rubber and other industrial chemicals.
Mechanism of Action
Mevalonic acid exerts its effects through the mevalonate pathway. It is converted to isopentenyl pyrophosphate, which serves as a building block for the synthesis of isoprenoids. The key enzyme in this pathway is 3-hydroxy-3-methylglutaryl-coenzyme A reductase, which catalyzes the conversion of hydroxymethylglutaryl-coenzyme A to this compound . This enzyme is the target of statins, which inhibit its activity to reduce cholesterol synthesis .
Comparison with Similar Compounds
Mevalonic acid is unique due to its role in the mevalonate pathway. Similar compounds include:
Isopentenyl pyrophosphate: A direct product of this compound.
Geranyl pyrophosphate: An intermediate in the synthesis of terpenoids.
Farnesyl pyrophosphate: Another intermediate in the isoprenoid biosynthesis pathway.
This compound stands out due to its central role in the biosynthesis of a wide range of essential biomolecules, making it a critical compound in both biological and industrial contexts .
Biological Activity
Mevalonic acid (MVA) is a crucial intermediate in the biosynthesis of cholesterol and other isoprenoids. Its biological activity extends beyond mere metabolic pathways; it plays significant roles in cell proliferation, membrane integrity, and potential therapeutic applications. This article explores the multifaceted biological activities of this compound, supported by case studies and relevant research findings.
1. Role in Cholesterol Biosynthesis
This compound is synthesized from 3-hydroxy-3-methylglutaryl-CoA (HMG-CoA) through the action of HMG-CoA reductase, the rate-limiting enzyme in cholesterol biosynthesis. This pathway is vital for producing cholesterol, which is essential for cell membrane structure and function. Research indicates that mevalonate-derived products are crucial for cell proliferation, as cells require cholesterol and other metabolites for growth and division .
2. Cellular Proliferation and Membrane Integrity
This compound has been implicated in various cellular processes, including:
- Cell Growth : Studies have shown that mevalonate is essential for the proliferation of different cell types. Inhibition of mevalonate synthesis can lead to reduced cell growth, demonstrating its role as a growth factor .
- Membrane Function : MVA contributes to the modification of membrane-associated proteins, including G proteins involved in signal transduction. This modification is critical for maintaining cellular functions such as osmolarity and endocytosis .
3.1 Mevalonic Acidemia
Mevalonic acidemia is a rare genetic disorder caused by mutations in the MVK gene, leading to elevated levels of this compound in the body. Clinical manifestations include developmental delays, dysmorphic features, and metabolic crises. A study involving 61 patients demonstrated that elevated urinary excretion of this compound could serve as a diagnostic marker for this condition, with high sensitivity (92%) and specificity (90%) .
Characteristic | MK-deficient Patients | MK-sufficient Patients with Elevated MVA | MK-sufficient Patients with Normal MVA |
---|---|---|---|
Number (n) | 13 | 12 | 48 |
Gender (M/F) | 5/8 | 3/9 | 24/24 |
Median Age (years) | 3.7 | 3.5 | 5.6 |
Median MVA Excretion (μmol/L) | 11 | 3.3 | 0.1 |
3.2 Pharmacological Applications
Recent studies have explored the potential of statins, which inhibit HMG-CoA reductase, to modulate mevalonate levels for therapeutic benefits:
- Cancer Treatment : Statins like simvastatin have shown promise in inhibiting tumor growth by blocking mevalonate synthesis. In vitro studies indicated that simvastatin could synergistically enhance the effects of chemotherapeutic agents on glioma cells .
- Skin Barrier Recovery : Topical application of this compound has been shown to enhance skin barrier recovery in aged mice by stimulating cholesterol synthesis and improving epidermal permeability barrier function .
The biological effects of this compound are mediated through several mechanisms:
- Cholesterol Synthesis Stimulation : MVA directly stimulates the activity of HMG-CoA reductase, enhancing cholesterol production necessary for cellular functions .
- Protein Modifications : Mevalonate-derived modifications are essential for the proper functioning of various proteins involved in cellular signaling and membrane integrity .
- Regulatory Effects on Gene Expression : The mevalonate pathway influences the expression of genes associated with lipid metabolism and cell cycle regulation .
Properties
IUPAC Name |
(3R)-3,5-dihydroxy-3-methylpentanoic acid | |
---|---|---|
Source | PubChem | |
URL | https://pubchem.ncbi.nlm.nih.gov | |
Description | Data deposited in or computed by PubChem | |
InChI |
InChI=1S/C6H12O4/c1-6(10,2-3-7)4-5(8)9/h7,10H,2-4H2,1H3,(H,8,9)/t6-/m1/s1 | |
Source | PubChem | |
URL | https://pubchem.ncbi.nlm.nih.gov | |
Description | Data deposited in or computed by PubChem | |
InChI Key |
KJTLQQUUPVSXIM-ZCFIWIBFSA-N | |
Source | PubChem | |
URL | https://pubchem.ncbi.nlm.nih.gov | |
Description | Data deposited in or computed by PubChem | |
Canonical SMILES |
CC(CCO)(CC(=O)O)O | |
Source | PubChem | |
URL | https://pubchem.ncbi.nlm.nih.gov | |
Description | Data deposited in or computed by PubChem | |
Isomeric SMILES |
C[C@@](CCO)(CC(=O)O)O | |
Source | PubChem | |
URL | https://pubchem.ncbi.nlm.nih.gov | |
Description | Data deposited in or computed by PubChem | |
Molecular Formula |
C6H12O4 | |
Source | PubChem | |
URL | https://pubchem.ncbi.nlm.nih.gov | |
Description | Data deposited in or computed by PubChem | |
DSSTOX Substance ID |
DTXSID801314151 | |
Record name | (R)-(-)-Mevalonic acid | |
Source | EPA DSSTox | |
URL | https://comptox.epa.gov/dashboard/DTXSID801314151 | |
Description | DSSTox provides a high quality public chemistry resource for supporting improved predictive toxicology. | |
Molecular Weight |
148.16 g/mol | |
Source | PubChem | |
URL | https://pubchem.ncbi.nlm.nih.gov | |
Description | Data deposited in or computed by PubChem | |
Physical Description |
Solid | |
Record name | Mevalonic acid | |
Source | Human Metabolome Database (HMDB) | |
URL | http://www.hmdb.ca/metabolites/HMDB0000227 | |
Description | The Human Metabolome Database (HMDB) is a freely available electronic database containing detailed information about small molecule metabolites found in the human body. | |
Explanation | HMDB is offered to the public as a freely available resource. Use and re-distribution of the data, in whole or in part, for commercial purposes requires explicit permission of the authors and explicit acknowledgment of the source material (HMDB) and the original publication (see the HMDB citing page). We ask that users who download significant portions of the database cite the HMDB paper in any resulting publications. | |
Solubility |
Very soluble in water, but also soluble in organic solvents, especially polar organic solvents. | |
Record name | MEVALONIC ACID | |
Source | Hazardous Substances Data Bank (HSDB) | |
URL | https://pubchem.ncbi.nlm.nih.gov/source/hsdb/7681 | |
Description | The Hazardous Substances Data Bank (HSDB) is a toxicology database that focuses on the toxicology of potentially hazardous chemicals. It provides information on human exposure, industrial hygiene, emergency handling procedures, environmental fate, regulatory requirements, nanomaterials, and related areas. The information in HSDB has been assessed by a Scientific Review Panel. | |
Color/Form |
Oily liquid | |
CAS No. |
17817-88-8, 150-97-0 | |
Record name | (R)-(-)-Mevalonic acid | |
Source | CAS Common Chemistry | |
URL | https://commonchemistry.cas.org/detail?cas_rn=17817-88-8 | |
Description | CAS Common Chemistry is an open community resource for accessing chemical information. Nearly 500,000 chemical substances from CAS REGISTRY cover areas of community interest, including common and frequently regulated chemicals, and those relevant to high school and undergraduate chemistry classes. This chemical information, curated by our expert scientists, is provided in alignment with our mission as a division of the American Chemical Society. | |
Explanation | The data from CAS Common Chemistry is provided under a CC-BY-NC 4.0 license, unless otherwise stated. | |
Record name | Mevalonic acid | |
Source | DrugBank | |
URL | https://www.drugbank.ca/drugs/DB03518 | |
Description | The DrugBank database is a unique bioinformatics and cheminformatics resource that combines detailed drug (i.e. chemical, pharmacological and pharmaceutical) data with comprehensive drug target (i.e. sequence, structure, and pathway) information. | |
Explanation | Creative Common's Attribution-NonCommercial 4.0 International License (http://creativecommons.org/licenses/by-nc/4.0/legalcode) | |
Record name | (R)-(-)-Mevalonic acid | |
Source | EPA DSSTox | |
URL | https://comptox.epa.gov/dashboard/DTXSID801314151 | |
Description | DSSTox provides a high quality public chemistry resource for supporting improved predictive toxicology. | |
Record name | MEVALONIC ACID | |
Source | Hazardous Substances Data Bank (HSDB) | |
URL | https://pubchem.ncbi.nlm.nih.gov/source/hsdb/7681 | |
Description | The Hazardous Substances Data Bank (HSDB) is a toxicology database that focuses on the toxicology of potentially hazardous chemicals. It provides information on human exposure, industrial hygiene, emergency handling procedures, environmental fate, regulatory requirements, nanomaterials, and related areas. The information in HSDB has been assessed by a Scientific Review Panel. | |
Record name | Mevalonic acid | |
Source | Human Metabolome Database (HMDB) | |
URL | http://www.hmdb.ca/metabolites/HMDB0000227 | |
Description | The Human Metabolome Database (HMDB) is a freely available electronic database containing detailed information about small molecule metabolites found in the human body. | |
Explanation | HMDB is offered to the public as a freely available resource. Use and re-distribution of the data, in whole or in part, for commercial purposes requires explicit permission of the authors and explicit acknowledgment of the source material (HMDB) and the original publication (see the HMDB citing page). We ask that users who download significant portions of the database cite the HMDB paper in any resulting publications. | |
Melting Point |
24 - 27 °C | |
Record name | Mevalonic acid | |
Source | Human Metabolome Database (HMDB) | |
URL | http://www.hmdb.ca/metabolites/HMDB0000227 | |
Description | The Human Metabolome Database (HMDB) is a freely available electronic database containing detailed information about small molecule metabolites found in the human body. | |
Explanation | HMDB is offered to the public as a freely available resource. Use and re-distribution of the data, in whole or in part, for commercial purposes requires explicit permission of the authors and explicit acknowledgment of the source material (HMDB) and the original publication (see the HMDB citing page). We ask that users who download significant portions of the database cite the HMDB paper in any resulting publications. | |
Retrosynthesis Analysis
AI-Powered Synthesis Planning: Our tool employs the Template_relevance Pistachio, Template_relevance Bkms_metabolic, Template_relevance Pistachio_ringbreaker, Template_relevance Reaxys, Template_relevance Reaxys_biocatalysis model, leveraging a vast database of chemical reactions to predict feasible synthetic routes.
One-Step Synthesis Focus: Specifically designed for one-step synthesis, it provides concise and direct routes for your target compounds, streamlining the synthesis process.
Accurate Predictions: Utilizing the extensive PISTACHIO, BKMS_METABOLIC, PISTACHIO_RINGBREAKER, REAXYS, REAXYS_BIOCATALYSIS database, our tool offers high-accuracy predictions, reflecting the latest in chemical research and data.
Strategy Settings
Precursor scoring | Relevance Heuristic |
---|---|
Min. plausibility | 0.01 |
Model | Template_relevance |
Template Set | Pistachio/Bkms_metabolic/Pistachio_ringbreaker/Reaxys/Reaxys_biocatalysis |
Top-N result to add to graph | 6 |
Feasible Synthetic Routes
Disclaimer and Information on In-Vitro Research Products
Please be aware that all articles and product information presented on BenchChem are intended solely for informational purposes. The products available for purchase on BenchChem are specifically designed for in-vitro studies, which are conducted outside of living organisms. In-vitro studies, derived from the Latin term "in glass," involve experiments performed in controlled laboratory settings using cells or tissues. It is important to note that these products are not categorized as medicines or drugs, and they have not received approval from the FDA for the prevention, treatment, or cure of any medical condition, ailment, or disease. We must emphasize that any form of bodily introduction of these products into humans or animals is strictly prohibited by law. It is essential to adhere to these guidelines to ensure compliance with legal and ethical standards in research and experimentation.