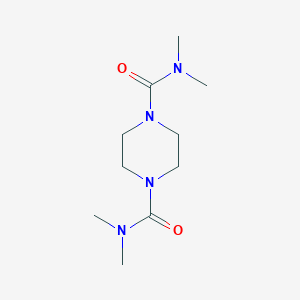
1-N,1-N,4-N,4-N-tetramethylpiperazine-1,4-dicarboxamide
- Click on QUICK INQUIRY to receive a quote from our team of experts.
- With the quality product at a COMPETITIVE price, you can focus more on your research.
Description
1-N,1-N,4-N,4-N-tetramethylpiperazine-1,4-dicarboxamide (TMD) is a chemical compound that has been widely used in scientific research. It is a cyclic amide that is structurally similar to piperazine, a common organic compound. TMD has been used in various fields of research, including medicinal chemistry, biochemistry, and pharmacology. In
Scientific Research Applications
Synthesis and Material Applications
1-N,1-N,4-N,4-N-tetramethylpiperazine-1,4-dicarboxamide and its derivatives have been synthesized for various applications in materials science. For instance, derivatives have been utilized in the synthesis of tetracarboxamide-tetra(1,4-dithiin) porphyrazine, demonstrating potential for use in simulated sunlight conditions for the degradation of organic dyes like methyl orange and RhB (Quan, Rong, & Ke-jian, 2015).
Medicinal Chemistry and Drug Design
In the realm of medicinal chemistry, N-methylpiperazine fragments, which are structurally related to 1-N,1-N,4-N,4-N-tetramethylpiperazine-1,4-dicarboxamide, have been incorporated into new carboxylic acid amides. These amides have been explored for their potential in drug development, highlighting the versatility of piperazine derivatives in synthesizing compounds with biological activity (Koroleva, Gusak, Ignatovich, & Ermolinskaya, 2011).
Catalysis and Chemical Reactions
The compound and its related derivatives have found use in catalysis and as intermediates in chemical reactions. For example, 1-amino-4-methylpiperazine, a compound related to 1-N,1-N,4-N,4-N-tetramethylpiperazine-1,4-dicarboxamide, has been utilized as an intermediate in the synthesis of medicinal drugs, showcasing the importance of piperazine derivatives in pharmaceutical synthesis (Kushakova, Kuznetsov, Chernobroviy, & Garabadgiu, 2004).
Imaging and Diagnostic Agents
Piperazine derivatives have also been explored as potential agents in medical imaging and diagnostics. For instance, a specific derivative was synthesized as a new potential PET agent for imaging of the IRAK4 enzyme in neuroinflammation, underscoring the role of these compounds in the development of diagnostic tools (Wang et al., 2018).
Polymer Science
In polymer science, poly(amido-amine)s containing piperazine units have been studied for their endosomolytic properties, which correlate with their physicochemical and biological properties. Such studies are crucial for the development of polymers with potential biomedical applications (Ferruti et al., 2000).
properties
IUPAC Name |
1-N,1-N,4-N,4-N-tetramethylpiperazine-1,4-dicarboxamide |
Source
|
---|---|---|
Source | PubChem | |
URL | https://pubchem.ncbi.nlm.nih.gov | |
Description | Data deposited in or computed by PubChem | |
InChI |
InChI=1S/C10H20N4O2/c1-11(2)9(15)13-5-7-14(8-6-13)10(16)12(3)4/h5-8H2,1-4H3 |
Source
|
Source | PubChem | |
URL | https://pubchem.ncbi.nlm.nih.gov | |
Description | Data deposited in or computed by PubChem | |
InChI Key |
IQHALCWAYMQEQI-UHFFFAOYSA-N |
Source
|
Source | PubChem | |
URL | https://pubchem.ncbi.nlm.nih.gov | |
Description | Data deposited in or computed by PubChem | |
Canonical SMILES |
CN(C)C(=O)N1CCN(CC1)C(=O)N(C)C |
Source
|
Source | PubChem | |
URL | https://pubchem.ncbi.nlm.nih.gov | |
Description | Data deposited in or computed by PubChem | |
Molecular Formula |
C10H20N4O2 |
Source
|
Source | PubChem | |
URL | https://pubchem.ncbi.nlm.nih.gov | |
Description | Data deposited in or computed by PubChem | |
Molecular Weight |
228.29 g/mol |
Source
|
Source | PubChem | |
URL | https://pubchem.ncbi.nlm.nih.gov | |
Description | Data deposited in or computed by PubChem | |
Product Name |
1-N,1-N,4-N,4-N-tetramethylpiperazine-1,4-dicarboxamide |
Q & A
Q1: What is the significance of the structural modification in N1,N1,N4,N4-tetramethylpiperazine-1,4-dicarboxamide compared to its parent piperazine in terms of larvicidal activity?
A1: While the research primarily focuses on the larvicidal activity of N1,N1,N4,N4-tetramethylpiperazine-1,4-dicarboxamide, it directly compares its efficacy to its parent compound, piperazine []. This comparison highlights the impact of the 1,4-dicarboxamide substitution on the molecule's ability to effectively target and disrupt the development of mosquito larvae. The study found that the addition of the 1,4-dicarboxamide group significantly enhances the larvicidal activity compared to the parent piperazine molecule [].
Q2: What are the observed effects of N1,N1,N4,N4-tetramethylpiperazine-1,4-dicarboxamide on the histological structure of mosquito larvae?
A2: The research observed significant damage to the histological structure of the midgut in third instar larvae of Aedes caspius and Culex pipiens exposed to N1,N1,N4,N4-tetramethylpiperazine-1,4-dicarboxamide []. The observed damage included cell destruction, vacuolization of epithelial cells, and cell dispersal within the midgut []. These findings suggest that the compound disrupts the normal cellular structure and function of the mosquito larvae's digestive system, ultimately leading to their death.
Disclaimer and Information on In-Vitro Research Products
Please be aware that all articles and product information presented on BenchChem are intended solely for informational purposes. The products available for purchase on BenchChem are specifically designed for in-vitro studies, which are conducted outside of living organisms. In-vitro studies, derived from the Latin term "in glass," involve experiments performed in controlled laboratory settings using cells or tissues. It is important to note that these products are not categorized as medicines or drugs, and they have not received approval from the FDA for the prevention, treatment, or cure of any medical condition, ailment, or disease. We must emphasize that any form of bodily introduction of these products into humans or animals is strictly prohibited by law. It is essential to adhere to these guidelines to ensure compliance with legal and ethical standards in research and experimentation.