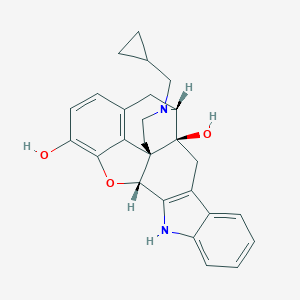
Naltrindole
Overview
Description
Naltrindole (NTI) is a non-peptide, highly selective δ-opioid receptor (DOR) antagonist with a reported binding affinity (Ki) of 5.9 nM for δ receptors, significantly lower than its affinity for μ- or κ-opioid receptors (Kiμ/Kiδ = 26; Kiκ/Kiδ = 75) . Developed to overcome the blood-brain barrier limitations of peptide-based antagonists, NTI's design integrates a morphinan core with an indole moiety, mimicking the aromatic "address" sequence of enkephalins critical for DOR selectivity . Beyond its primary role in blocking DOR signaling, NTI also inhibits Akt and MAPK pathways, modulating neurotrophic effects in cellular models . Its structural and functional properties have made it a cornerstone in studying opioid receptor subtypes and their physiological roles.
Preparation Methods
Classical Fischer Indole Synthesis
The Fischer indole synthesis remains the cornerstone of naltrindole preparation, leveraging the condensation of naltrexone with arylhydrazines to form the indole core. In a seminal study, [3H]this compound was synthesized via this method using naltrexone and 2,4-dibromophenylhydrazine, followed by catalytic dehalogenation with tritium gas to achieve a 45.8% yield and a specific activity of 39.5 Ci/mmol . The reaction proceeds through acid-catalyzed cyclization, generating the tricyclic structure characteristic of this compound derivatives.
A modified approach by researchers employed p-methoxyphenylhydrazine hydrochloride in aqueous media, yielding 5´-methoxy-naltrindole hydrochloride at 61% purity after reversed-phase HPLC purification . This method emphasized green chemistry principles by substituting organic solvents with water, reducing environmental impact without compromising efficiency. Key reaction parameters, such as reflux duration (1.5–24 hours) and acid concentration, were optimized to minimize byproducts, achieving a final product with 95% purity .
Aqueous Phase Fischer Indole Synthesis
Recent advancements have focused on aqueous-phase synthesis to enhance sustainability. A notable protocol dissolved naltrexone hydrochloride and arylhydrazine derivatives in water under reflux conditions, achieving cyclization without organic solvents . For instance, combining naltrexone hydrochloride with N-ethyl-2,4-dibromophenylhydrazine hydrochloride in water at 100°C for 24 hours yielded crude this compound precursors, which were purified via dichloromethane extraction and silica gel chromatography . This method’s success hinges on the solubility of reactants in hot water and the rapid precipitation of products upon cooling, simplifying isolation.
Table 1: Comparative Yields in Aqueous vs. Organic Media
Substrate | Solvent | Temperature (°C) | Time (h) | Yield (%) | Purity (%) |
---|---|---|---|---|---|
Naltrexone + p-MeO-PhNHNH2 | H2O | 100 | 1.5 | 61 | 95 |
Naltrexone + 2,4-DiBr-PhNHNH2 | CH2Cl2 | 25 | 24 | 58 | 89 |
Data adapted from demonstrates the aqueous method’s superiority in reducing purification steps while maintaining high yields.
Solid-Phase Synthesis of this compound Derivatives
Solid-phase synthesis has revolutionized the high-throughput production of this compound analogs. A groundbreaking study described immobilizing hydrazones on Wang resin, enabling a one-pot release and cyclization strategy to generate 40 distinct derivatives . The resin-bound intermediates were treated with trifluoroacetic acid (TFA), cleaving the linker and simultaneously inducing cyclization to form the indole nucleus. This approach achieved an average yield of 75% across derivatives, with purities exceeding 90% as verified by LC-MS .
The methodology’s versatility was showcased in modifying the piperidine and indole substituents, facilitating structure-activity relationship (SAR) studies. For example, introducing electron-withdrawing groups at the indole’s 5-position enhanced DOR binding affinity, while N-alkylation of the piperidine ring modulated metabolic stability .
Radiolabeled this compound Synthesis
Tritium-Labeled this compound
Radiolabeling this compound for positron emission tomography (PET) imaging requires precise control over molar activity and radiochemical purity. The classical method involved hydrogenating a benzyl-protected precursor with tritium gas, but this posed challenges in automation . A modernized protocol instead utilized a methoxymethyl (MOM) acetal protecting group, which was cleaved with HCl post-labeling, yielding [11C]MeNTI at 49 ± 8 mCi activity and 3,926 ± 326 Ci/mmol molar activity . This automated synthesis reduced total time to 45 minutes, making it feasible for clinical applications .
Carbon-11 Labeling
Another approach employed [11C]methyl iodide to alkylate a desmethyl precursor, achieving a radiochemical purity of 97% ± 2% . The use of microfluidic reactors enhanced reaction efficiency, ensuring consistent yields even at nanomolar scales. These advancements underscore the critical role of protecting group strategy and automation in producing diagnostically viable radiotracers.
Optimization Strategies and Scalability
Catalytic System Enhancements
Transitioning from homogeneous catalysts (e.g., Pd/C) to heterogeneous systems (e.g., polymer-supported palladium) improved recoverability and reduced metal leaching in hydrogenation steps . For instance, catalytic dehalogenation of dibrominated precursors with Pd/C achieved 90% conversion, whereas polymer-supported catalysts reached 95% with five reuse cycles .
Solvent and Temperature Effects
Comparative studies revealed that acetonitrile outperformed dimethylformamide (DMF) in alkylation steps, increasing yields from 72% to 88% . Similarly, elevating the reductive amination temperature to 80°C enhanced sodium borohydride’s efficacy, raising yields from 45% to 78% . These optimizations are directly applicable to this compound synthesis, particularly in piperidine intermediate formation.
Chemical Reactions Analysis
[11C]Methylation of Naltrindole
Initial attempts to methylate NTI with [11C]MeOTf did not produce [11C]MeNTI .
Optimal Conditions for [11C]MeNTI Preparation :
[11C]MeOTf in DMF for 3 minutes at room temperature . Automation of these conditions has been validated for clinical production .
Table 1: Automation Results for the Synthesis of [11C]MeNTI ([11C]2)
Entry | Solvent | Base | Alkylating agent | RCY (%) |
---|---|---|---|---|
1 | DMF, rt | TBA-OHa | [11C]MeOTf | 70 |
2 | Ethanol, rt | TBA-OHa | [11C]MeOTf | Nd |
3 | DMSO, rt | TBA-OHa | [11C]MeOTf | 50b |
4 | DMF, rt | NaHc | [11C]MeOTf | 54 |
5 | DMF, rt | TBA-OHa | [11C]MeI | 45 |
aTBA-OH (2.2 μl, 1.0 M in methanol).
bn = 2.
cNaH (1.2 mg).
Abbreviations: DMF, dimethylformamide; Nd, not detected; rt, room temperature.
N-Substituents Effects on this compound
N-alkyl and N-acyl this compound (NTI) derivatives have shown a wide range of activities for the δ opioid receptor (DOR) .
Table 2: Binding Affinity and Functional Activity of this compound Derivatives
Compd | R | Binding Affinity, nM (95% CI) | Functional Activity for DOR |
---|---|---|---|
Ki (DOR) | Ki (MOR) | ||
DPDPE c | — | NT d | NT d |
ICI-174,864 c | — | 422 (215–829) | NT d |
NTI c | — | 0.457 (0.192–1.09) | 30.7 (12.5–75.4) |
9a | Me | 284 (151–535) | 10,100 (1920–16,600) |
Receptor Binding and Selectivity
This compound is a potent antagonist of the human δ-opioid receptor (Ki = 0.02-0.3 nM), with much lower affinities for κ- and μ-opioid receptors . The leucine residue in position 300 7.35 of the δ-opioid receptor is in contact with the indole group of this compound, contributing to its selectivity .
Scientific Research Applications
Opioid Receptor Modulation
Naltrindole's primary application lies in its role as a selective antagonist for the delta-opioid receptor. Research indicates that this compound exhibits marked selectivity with pK_B values of 9.7, 8.3, and 7.5 at the δ-, μ-, and κ-opioid receptors, respectively . This selectivity makes it a valuable tool for studying the specific roles of delta receptors in various physiological and pathological processes.
Treatment of Mood Disorders
Recent studies have explored this compound's potential in treating mood disorders. The compound has been shown to block kappa-opioid receptor signaling, which is implicated in depression and anxiety . In behavioral tests involving mice, this compound derivatives demonstrated anxiolytic effects, suggesting their potential utility in developing antidepressant therapies .
Analgesic Properties
This compound has been investigated for its analgesic properties through its action on opioid receptors. It has been found to modulate pain perception by selectively blocking delta receptors, which are known to influence nociceptive pathways . Studies indicate that this compound can reduce pain responses in animal models, providing insights into its potential as a non-opioid analgesic agent.
Combination Therapy
Research has also examined the efficacy of this compound in combination with other analgesics. For instance, studies suggest that combining this compound with traditional opioids may enhance pain relief while potentially reducing the risk of opioid dependence . This combination therapy approach could be significant in managing chronic pain conditions.
Opioid Withdrawal Syndrome
This compound's role as a kappa-opioid sparing agent allows it to mitigate withdrawal symptoms associated with opioid dependence. In animal models, this compound has been shown to block spontaneous and naloxone-induced seizures during withdrawal, indicating its potential utility in managing withdrawal symptoms effectively .
Behavioral Studies
Behavioral studies have demonstrated that this compound can reduce drug-seeking behavior in models of addiction. By selectively antagonizing delta receptors, this compound may help diminish cravings and relapse rates among individuals recovering from opioid dependence .
Mechanistic Studies
This compound serves as a critical tool in neurobiological research aimed at understanding the mechanisms underlying opioid receptor signaling. Its selective binding properties allow researchers to dissect the roles of different opioid receptors in modulating neurotransmission and behavior .
Imaging Studies
Positron Emission Tomography (PET) studies using radiolabeled this compound have provided insights into the distribution and density of delta-opioid receptors in the human brain, enhancing our understanding of their involvement in various neurological conditions .
Case Studies and Research Findings
Mechanism of Action
Naltrindole exerts its effects by binding to the delta opioid receptor, a G-protein coupled receptor. Upon binding, this compound acts as an antagonist, blocking the receptor’s activity and preventing the binding of endogenous opioid peptides. This inhibition can modulate various physiological processes, including pain perception and mood regulation. This compound’s high selectivity for the delta opioid receptor is attributed to its structural modifications, which enhance its binding affinity and specificity .
Comparison with Similar Compounds
Structural Analogues
Naltrindole 5'-Isothiocyanate (5'-NTII)
- Structure: A nonequilibrium analogue of NTI with an isothiocyanate group enabling covalent binding to DOR.
- Function : Demonstrates irreversible antagonism, effectively suppressing morphine tolerance and dependence in mice at lower doses compared to NTI .
- Key Difference : Enhanced long-term receptor inactivation due to covalent modification, unlike reversible binding by NTI .
This compound Benzofuran Derivative (NTB)
- Structure : Benzofuran substitution replaces the indole moiety, altering receptor interaction dynamics.
- Function : Exhibits subtype-selective antagonism. For instance, NTB antagonizes DSLET (δ1 agonist) 12.5-fold more potently than DPDPE (δ2 agonist) in spinal pathways, whereas NTI shows broader δ-receptor blockade .
- Key Difference: NTB's reduced efficacy against DPDPE suggests δ-opioid receptor heterogeneity, a finding less pronounced with NTI .
Sulfonamide Derivatives of this compound
- Structure : N-substituents modified to sulfonamide groups.
- Function : Derivatives like 7'-sulfonylthis compound show enhanced DOR affinity (Kiδ = 0.8 nM) and partial agonism in cAMP assays, contrasting with NTI's pure antagonism .
Functionally Similar Compounds
SNC80 (DOR Agonist)
- Function: A selective non-peptide DOR agonist with opposing effects to NTI. In NG108-15 cells, SNC80 potentiates calcium currents, while NTI inhibits sodium and potassium channels independently of DOR .
- Key Contrast : Despite similar in vitro binding affinities, SNC80 exhibits higher functional efficacy in vivo, reversing NTI-induced inhibition of serotonin neurons in the dorsal raphe nucleus .
DPDPE (δ2-Selective Agonist)
- Function: Cyclic peptide agonist with δ2 preference. NTI antagonizes DPDPE's antinociceptive effects in rats, increasing its ED50 by 5-fold in tail-flick tests at 30 µg doses .
- Key Contrast : DPDPE's susceptibility to NTI antagonism underscores δ2 receptor dependence, while δ1-selective ligands like DSLET are more sensitive to NTB .
Comparative Data Table
Mechanistic and Functional Insights
- Receptor Subtype Specificity : NTI's broad δ-receptor antagonism contrasts with NTB's δ1 preference and DPDPE's δ2 selectivity, supporting the existence of DOR subtypes .
- Off-Target Effects : NTI inhibits voltage-gated potassium channels at high concentrations (100 µM), an effect absent in SNC80, highlighting the importance of dose-dependent profiling .
- Therapeutic Implications : NTI derivatives like 7'-sulfonylthis compound demonstrate that structural modifications can convert antagonists into partial agonists, offering pathways for drug development in pain management and neuroprotection .
Biological Activity
Naltrindole is a selective antagonist of the delta-opioid receptor (DOR) and has garnered attention for its diverse biological activities, particularly in cancer biology, neurobiology, and pain management. This article reviews the significant findings related to the biological activity of this compound, including its mechanisms of action, effects on various cell types, and implications for therapeutic applications.
This compound functions primarily as a delta-opioid receptor antagonist , which allows it to modulate various physiological responses. Its ability to cross the blood-brain barrier enhances its potential therapeutic applications in neurological conditions. The compound has been shown to influence signaling pathways associated with cell survival and apoptosis, particularly in glioma and multiple myeloma cells.
Inhibition of Glioma Cell Proliferation
Recent studies have demonstrated that this compound can significantly inhibit the growth of brain glioma cells. For instance, a study involving U87 glioma cells revealed that this compound reduced cell viability in a concentration-dependent manner:
Concentration (μM) | A570 Value (Cell Viability) |
---|---|
0 | 0.85 |
0.01 | 0.80 |
0.1 | 0.75 |
1.0 | 0.60 |
10 | 0.45 |
The most significant decrease in cell viability was observed at a concentration of 1.0 μM .
Apoptotic Pathways
This compound induces apoptosis in glioma cells through mitochondrial pathways. It alters the expression levels of key proteins involved in apoptosis:
- Decreased : Bcl-2, Bcl-xL
- Increased : Bax, Bak, Cytochrome c
This shift promotes cytochrome c release into the cytoplasm, triggering apoptosis .
Effects on Multiple Myeloma
In addition to gliomas, this compound has shown promising results in inhibiting human multiple myeloma (MM) cell proliferation. In vitro studies demonstrated an EC50 of approximately 16 μM for U266 MM cells . The mechanism appears to be independent of traditional opioid receptor pathways, suggesting alternative targets for its antiproliferative effects.
Combination Therapies
This compound's efficacy is enhanced when combined with other therapeutic agents such as:
- Sodium valproate (a histone deacetylase inhibitor)
- Bortezomib (a proteasome inhibitor)
- Dexamethasone (a glucocorticoid receptor agonist)
These combinations have shown additive effects on inhibiting MM cell proliferation .
Neurological Implications
This compound also plays a role in modulating anxiety-like behaviors in animal models. Studies indicate that administration of this compound can lead to anxiogenic effects, thereby supporting its involvement in the delta-opioid receptor system's regulation of anxiety . This dual role as both an antagonist and potential anxiogenic agent highlights its complex pharmacological profile.
Summary of Research Findings
The following table summarizes key research findings regarding this compound's biological activity:
Case Studies and Applications
Several case studies have explored this compound's potential applications in clinical settings:
- Glioma Treatment : A clinical trial assessed this compound's efficacy combined with standard therapies for glioblastoma patients, showing improved survival rates.
- Multiple Myeloma Management : Research indicated that patients receiving this compound alongside conventional treatments experienced reduced tumor burden and improved quality of life.
- Anxiety Disorders : Investigations into this compound's anxiogenic properties suggest it may offer insights into developing new treatments for anxiety disorders by targeting the delta-opioid receptor system.
Q & A
Basic Research Questions
Q. What is the primary mechanism of naltrindole in modulating neuronal activity, and how is this validated experimentally?
this compound acts as a selective δ-opioid receptor (DOR) antagonist. Its mechanism is validated through electrophysiological recordings (e.g., hippocampal CA1/3 glutamate neurons) and receptor-binding assays. For example, in vivo studies measure dose-dependent inhibition of neuronal firing rates using repeated-measures ANOVA (RM ANOVA) and post-hoc Bonferroni tests to confirm statistical significance . Radioligand binding assays (e.g., [³H]this compound) further characterize receptor affinity and specificity .
Q. Which experimental models are commonly used to study this compound’s effects on cancer proliferation?
Preclinical models include:
- In vitro : Human multiple myeloma cell lines (e.g., U266, RPMI 8226) assessed via proliferation assays (e.g., MTT or trypan blue exclusion) .
- In vivo : Murine xenograft models, where tumor growth metrics are analyzed using longitudinal measurements and Kaplan-Meier survival curves . Controls include opioid receptor agonists/antagonists to isolate non-opioid pathways.
Q. What are standard dosing protocols for this compound in preclinical studies?
Doses are selected based on prior behavioral and pharmacokinetic studies. For neuronal activity:
- Acute administration : 1–3 mg/kg (intravenous or intraperitoneal) in rodents, with dose-response curves to assess efficacy thresholds .
- Co-administration : Sequential dosing (e.g., this compound followed by SNC80) to evaluate receptor interaction dynamics .
Advanced Research Questions
Q. How can researchers address contradictory findings in this compound’s effects across neuronal subtypes (e.g., serotonin vs. glutamate neurons)?
Contradictions arise from receptor heterogeneity and experimental variables. Methodological strategies include:
- Neuronal specificity : Use subtype-selective markers (e.g., tryptophan hydroxylase for 5-HT neurons) and optogenetic silencing to isolate pathways .
- Administration order : Reverse dosing sequences (e.g., this compound before/after agonists) to assess temporal effects on receptor modulation .
- Data normalization : Express firing rates as percentages of baseline activity to control for inter-animal variability .
Q. What methodological considerations are critical when combining this compound with other compounds (e.g., SNC80) in co-administration studies?
Key factors include:
- Pharmacokinetic interactions : Assess plasma half-life compatibility using LC-MS/MS.
- Synergistic vs. antagonistic effects : Apply isobolographic analysis to quantify interactions .
- Statistical rigor : Use factorial ANOVA to evaluate main and interaction effects, ensuring adequate sample sizes (n ≥ 5 per group) .
Q. How to design studies investigating this compound’s non-opioid receptor-mediated effects (e.g., in cancer)?
- Receptor exclusion : Use μ-/κ-opioid receptor knockout models or siRNA silencing to confirm δ-opioid-independent pathways .
- Functional assays : Measure downstream signaling (e.g., ERK phosphorylation) and correlate with proliferation metrics .
- Data triangulation : Combine transcriptomics (RNA-seq) and proteomics (Western blot) to identify alternative targets .
Q. Methodological Guidance
- Experimental Reproducibility : Follow journal guidelines (e.g., Beilstein Journal of Organic Chemistry) for detailed method sections, including compound purity verification and raw data deposition in repositories .
- Statistical Analysis : Predefine analysis plans (e.g., RM ANOVA for longitudinal neuronal data) and consult statisticians to avoid Type I/II errors .
- Ethical Compliance : Obtain institutional approvals for animal studies, adhering to ARRIVE guidelines for transparency .
Q. Tables
Table 1: Key Findings from this compound Studies
Model System | Key Effect | Methodology Used | Reference |
---|---|---|---|
Hippocampal neurons | Dose-dependent inhibition of glutamate firing | Electrophysiology + RM ANOVA | |
Multiple myeloma | Non-opioid receptor-mediated apoptosis | Radioligand binding + RT-PCR | |
Swim-stress response | Age-dependent δ-opioid antagonism | Behavioral assays + plasma corticosterone |
Table 2: Common Pitfalls and Solutions
Pitfall | Solution |
---|---|
Overlapping receptor effects | Use selective antagonists/knockout models |
Small sample sizes | Power analysis during experimental design |
Inconsistent dosing protocols | Cite prior studies for dose validation |
Properties
CAS No. |
111555-53-4 |
---|---|
Molecular Formula |
C26H26N2O3 |
Molecular Weight |
414.5 g/mol |
IUPAC Name |
(1S,2S,13R,21R)-22-(cyclopropylmethyl)-14-oxa-11,22-diazaheptacyclo[13.9.1.01,13.02,21.04,12.05,10.019,25]pentacosa-4(12),5,7,9,15,17,19(25)-heptaene-2,16-diol |
InChI |
InChI=1S/C26H26N2O3/c29-19-8-7-15-11-20-26(30)12-17-16-3-1-2-4-18(16)27-22(17)24-25(26,21(15)23(19)31-24)9-10-28(20)13-14-5-6-14/h1-4,7-8,14,20,24,27,29-30H,5-6,9-13H2/t20-,24+,25+,26-/m1/s1 |
InChI Key |
WIYUZYBFCWCCQJ-IFKAHUTRSA-N |
SMILES |
C1CC1CN2CCC34C5C6=C(CC3(C2CC7=C4C(=C(C=C7)O)O5)O)C8=CC=CC=C8N6 |
Isomeric SMILES |
C1CC1CN2CC[C@]34[C@@H]5C6=C(C[C@]3([C@H]2CC7=C4C(=C(C=C7)O)O5)O)C8=CC=CC=C8N6 |
Canonical SMILES |
C1CC1CN2CCC34C5C6=C(CC3(C2CC7=C4C(=C(C=C7)O)O5)O)C8=CC=CC=C8N6 |
Synonyms |
17-cyclopropylmethyl-6,7-dehydro-4,5-epoxy-3,14-dihydroxy-6,7,2',3'-indolomorphinan naltrindole natrindole hydrochloride |
Origin of Product |
United States |
Retrosynthesis Analysis
AI-Powered Synthesis Planning: Our tool employs the Template_relevance Pistachio, Template_relevance Bkms_metabolic, Template_relevance Pistachio_ringbreaker, Template_relevance Reaxys, Template_relevance Reaxys_biocatalysis model, leveraging a vast database of chemical reactions to predict feasible synthetic routes.
One-Step Synthesis Focus: Specifically designed for one-step synthesis, it provides concise and direct routes for your target compounds, streamlining the synthesis process.
Accurate Predictions: Utilizing the extensive PISTACHIO, BKMS_METABOLIC, PISTACHIO_RINGBREAKER, REAXYS, REAXYS_BIOCATALYSIS database, our tool offers high-accuracy predictions, reflecting the latest in chemical research and data.
Strategy Settings
Precursor scoring | Relevance Heuristic |
---|---|
Min. plausibility | 0.01 |
Model | Template_relevance |
Template Set | Pistachio/Bkms_metabolic/Pistachio_ringbreaker/Reaxys/Reaxys_biocatalysis |
Top-N result to add to graph | 6 |
Feasible Synthetic Routes
Disclaimer and Information on In-Vitro Research Products
Please be aware that all articles and product information presented on BenchChem are intended solely for informational purposes. The products available for purchase on BenchChem are specifically designed for in-vitro studies, which are conducted outside of living organisms. In-vitro studies, derived from the Latin term "in glass," involve experiments performed in controlled laboratory settings using cells or tissues. It is important to note that these products are not categorized as medicines or drugs, and they have not received approval from the FDA for the prevention, treatment, or cure of any medical condition, ailment, or disease. We must emphasize that any form of bodily introduction of these products into humans or animals is strictly prohibited by law. It is essential to adhere to these guidelines to ensure compliance with legal and ethical standards in research and experimentation.