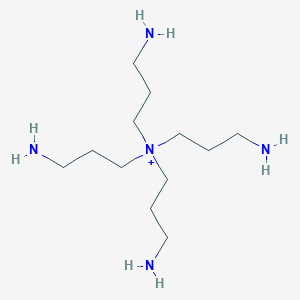
Tetrakis(3-aminopropyl)ammonium
Overview
Description
Tetrakis(3-aminopropyl)ammonium is a branched polyamine compound often found in cells of extreme thermophiles and hyperthermophiles. These organisms belong to both Bacteria and Archaea domains and thrive in extreme temperatures. The compound plays a crucial role in stabilizing nucleic acids and proteins under such conditions .
Preparation Methods
Synthetic Routes and Reaction Conditions
The synthesis of tetrakis(3-aminopropyl)ammonium involves the reaction of ammonia with 3-aminopropylamine under controlled conditions. The process typically requires a catalyst and is carried out at elevated temperatures to ensure complete reaction . The original method for the synthesis has been modified to improve yield and efficiency .
Industrial Production Methods
Industrial production of this compound follows similar synthetic routes but on a larger scale. The process involves the use of high-pressure reactors and continuous flow systems to maintain optimal reaction conditions and maximize yield .
Chemical Reactions Analysis
Types of Reactions
Tetrakis(3-aminopropyl)ammonium undergoes various chemical reactions, including:
Oxidation: The compound can be oxidized to form corresponding amine oxides.
Reduction: It can be reduced to form simpler amines.
Substitution: The amino groups can participate in substitution reactions with various electrophiles.
Common Reagents and Conditions
Common reagents used in these reactions include oxidizing agents like hydrogen peroxide for oxidation, reducing agents like lithium aluminum hydride for reduction, and electrophiles like alkyl halides for substitution reactions .
Major Products
The major products formed from these reactions include amine oxides, simpler amines, and substituted polyamines .
Scientific Research Applications
Tetrakis(3-aminopropyl)ammonium has a wide range of scientific research applications:
Mechanism of Action
Tetrakis(3-aminopropyl)ammonium stabilizes nucleic acids by binding electrostatically to the negatively charged phosphate groups within DNA and RNA. This binding prevents thermal denaturation and maintains the structural integrity of nucleic acids under extreme conditions . The compound also enhances the activity of cell-free translation systems by stabilizing the ternary complex between ribosomes, messenger RNA, and transfer RNA .
Comparison with Similar Compounds
Similar Compounds
- Caldopentamine
- Caldohexamine
- N4-aminopropylspermidine
- N4-bis(aminopropyl)spermidine
Uniqueness
Tetrakis(3-aminopropyl)ammonium is unique due to its branched structure, which provides enhanced stability to nucleic acids and proteins compared to linear polyamines. This makes it particularly effective in extreme conditions where other polyamines may not perform as well .
Biological Activity
Tetrakis(3-aminopropyl)ammonium (TAA) is a branched polyamine compound with significant biological activity, particularly in stabilizing nucleic acids and proteins under extreme conditions. This article explores its mechanisms of action, applications in various fields, and relevant research findings.
Overview of this compound
TAA is characterized by its quaternary ammonium structure, which allows it to interact electrostatically with negatively charged phosphate groups in nucleic acids. This interaction is crucial for maintaining the structural integrity of DNA and RNA, especially in thermophilic organisms that thrive in high-temperature environments .
The primary mechanism through which TAA exerts its biological activity involves:
- Electrostatic Binding : TAA binds to the phosphate backbone of nucleic acids, preventing thermal denaturation and stabilizing their structures during high-temperature conditions.
- Stabilization of RNA : Research indicates that TAA is particularly effective in stabilizing single-stranded RNA (ssRNA) and tRNA, outperforming other polyamines in this regard .
Comparative Stability Analysis
The stabilization effects of TAA compared to other polyamines can be summarized in the following table:
Polyamine | Stabilization of dsDNA | Stabilization of ssRNA |
---|---|---|
This compound | Moderate | High |
Caldopentamine | High | Moderate |
Thermospermine | Moderate | Low |
This table illustrates that while linear polyamines like caldopentamine are more effective at stabilizing double-stranded DNA (dsDNA), TAA excels in stabilizing ssRNA structures .
Case Studies and Research Findings
- Thermal Denaturation Studies : A study utilizing differential scanning calorimetry demonstrated that the melting temperature () of dsDNA increased with higher concentrations of TAA. This indicates a strong correlation between TAA concentration and its stabilizing effect on nucleic acids .
- Poly-ion Complex Formation : TAA has been utilized to form poly-ion complexes with heparin, which allows for sustained release applications in medical therapies. The stability and sticky nature of these complexes enhance their potential for drug delivery systems .
- Role in Extreme Environments : Research has shown that TAA plays a critical role in the survival of extreme thermophiles by stabilizing essential biomolecules under conditions that would typically lead to denaturation or degradation .
Applications
TAA's unique properties have led to its application across various fields:
Properties
IUPAC Name |
tetrakis(3-aminopropyl)azanium | |
---|---|---|
Source | PubChem | |
URL | https://pubchem.ncbi.nlm.nih.gov | |
Description | Data deposited in or computed by PubChem | |
InChI |
InChI=1S/C12H32N5/c13-5-1-9-17(10-2-6-14,11-3-7-15)12-4-8-16/h1-16H2/q+1 | |
Source | PubChem | |
URL | https://pubchem.ncbi.nlm.nih.gov | |
Description | Data deposited in or computed by PubChem | |
InChI Key |
XJXHSXSKNSIRGP-UHFFFAOYSA-N | |
Source | PubChem | |
URL | https://pubchem.ncbi.nlm.nih.gov | |
Description | Data deposited in or computed by PubChem | |
Canonical SMILES |
C(CN)C[N+](CCCN)(CCCN)CCCN | |
Source | PubChem | |
URL | https://pubchem.ncbi.nlm.nih.gov | |
Description | Data deposited in or computed by PubChem | |
Molecular Formula |
C12H32N5+ | |
Source | PubChem | |
URL | https://pubchem.ncbi.nlm.nih.gov | |
Description | Data deposited in or computed by PubChem | |
DSSTOX Substance ID |
DTXSID80149542 | |
Record name | Tetrakis(3-aminopropyl)ammonium | |
Source | EPA DSSTox | |
URL | https://comptox.epa.gov/dashboard/DTXSID80149542 | |
Description | DSSTox provides a high quality public chemistry resource for supporting improved predictive toxicology. | |
Molecular Weight |
246.42 g/mol | |
Source | PubChem | |
URL | https://pubchem.ncbi.nlm.nih.gov | |
Description | Data deposited in or computed by PubChem | |
CAS No. |
111216-37-6 | |
Record name | Tetrakis(3-aminopropyl)ammonium | |
Source | ChemIDplus | |
URL | https://pubchem.ncbi.nlm.nih.gov/substance/?source=chemidplus&sourceid=0111216376 | |
Description | ChemIDplus is a free, web search system that provides access to the structure and nomenclature authority files used for the identification of chemical substances cited in National Library of Medicine (NLM) databases, including the TOXNET system. | |
Record name | Tetrakis(3-aminopropyl)ammonium | |
Source | EPA DSSTox | |
URL | https://comptox.epa.gov/dashboard/DTXSID80149542 | |
Description | DSSTox provides a high quality public chemistry resource for supporting improved predictive toxicology. | |
Retrosynthesis Analysis
AI-Powered Synthesis Planning: Our tool employs the Template_relevance Pistachio, Template_relevance Bkms_metabolic, Template_relevance Pistachio_ringbreaker, Template_relevance Reaxys, Template_relevance Reaxys_biocatalysis model, leveraging a vast database of chemical reactions to predict feasible synthetic routes.
One-Step Synthesis Focus: Specifically designed for one-step synthesis, it provides concise and direct routes for your target compounds, streamlining the synthesis process.
Accurate Predictions: Utilizing the extensive PISTACHIO, BKMS_METABOLIC, PISTACHIO_RINGBREAKER, REAXYS, REAXYS_BIOCATALYSIS database, our tool offers high-accuracy predictions, reflecting the latest in chemical research and data.
Strategy Settings
Precursor scoring | Relevance Heuristic |
---|---|
Min. plausibility | 0.01 |
Model | Template_relevance |
Template Set | Pistachio/Bkms_metabolic/Pistachio_ringbreaker/Reaxys/Reaxys_biocatalysis |
Top-N result to add to graph | 6 |
Feasible Synthetic Routes
Disclaimer and Information on In-Vitro Research Products
Please be aware that all articles and product information presented on BenchChem are intended solely for informational purposes. The products available for purchase on BenchChem are specifically designed for in-vitro studies, which are conducted outside of living organisms. In-vitro studies, derived from the Latin term "in glass," involve experiments performed in controlled laboratory settings using cells or tissues. It is important to note that these products are not categorized as medicines or drugs, and they have not received approval from the FDA for the prevention, treatment, or cure of any medical condition, ailment, or disease. We must emphasize that any form of bodily introduction of these products into humans or animals is strictly prohibited by law. It is essential to adhere to these guidelines to ensure compliance with legal and ethical standards in research and experimentation.