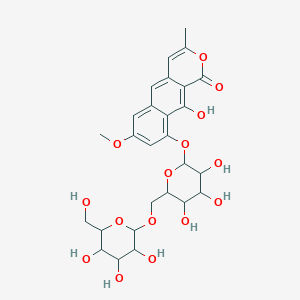
Cassiaside C
Overview
Description
Cassiaside C (C₂₇H₃₂O₁₅, molecular weight: 596.53 g/mol) is a naphthopyrone glycoside isolated from Cassiae semen (seeds of Cassia tora L.), a traditional medicinal herb widely used in East Asia . It exhibits multiple pharmacological activities, including:
- Anti-inflammatory effects: Inhibition of M1 macrophage polarization via suppression of the PI3K/AKT/mTORC1 signaling pathway and downregulation of glycolysis-related proteins (HIF-1α, PDK1, LDHA) .
- Neuroprotective properties: Potential anti-Alzheimer’s activity via BACE1 (β-secretase) inhibition (IC₅₀ = 4.45 μM) .
Its mechanism in macrophage modulation involves reducing pro-inflammatory cytokines (TNF-α, IL-1β, IL-6) and metabolic reprogramming from glycolysis to oxidative phosphorylation .
Preparation Methods
Synthetic Routes and Reaction Conditions: Cassiaside C can be synthesized through various chemical reactions involving the glycosylation of toralactone. The synthetic route typically involves the use of glycosyl donors and acceptors under specific reaction conditions to form the glycosidic bond. The reaction conditions often include the use of catalysts, solvents, and controlled temperatures to achieve the desired product.
Industrial Production Methods: Industrial production of this compound involves the extraction and purification of the compound from the seeds of Cassia obtusifolia. The process includes steps such as solvent extraction, filtration, and chromatographic techniques to isolate and purify this compound. The use of advanced extraction technologies ensures high yield and purity of the compound.
Chemical Reactions Analysis
Naphthopyrone Core Construction
-
Condensation Reaction : The naphtho-α-pyrone backbone (toralactone) was synthesized via a condensation reaction between orsellinate (8 ) and pyrone (9 ) using lithium diisopropylamide (LDA) .
Glycosylation Steps
-
Glycosyl Donors : Trifluoroacetimidates (6f , 6g ) and trichloroacetimidates were employed for sequential glycosylation.
-
Conditions :
Glycosylation Step | Donor | Conditions | Yield |
---|---|---|---|
Primary glycosylation | Trifluoroacetimidate | 0.05 equiv TMSOTf, 6.0 equiv donor | 85–90% |
Secondary glycosylation | Trichloroacetimidate | 0.3 equiv TMSOTf, 6.0 equiv donor | 78–82% |
Naphthol Hydroxyl Group
-
The phenolic hydroxyl group at the naphthopyrone core demonstrates low nucleophilicity, requiring aggressive glycosylation conditions (e.g., excess donor, strong Lewis acids) .
Glycosidic Bond Stability
-
Cassiaside C’s β-D-gentiobioside linkages are resistant to hydrolysis under mild acidic or enzymatic conditions due to the steric protection of the glycosidic bonds .
Biotransformation and Metabolic Pathways
While direct chemical reactions in biological systems are less documented, this compound undergoes metabolic modifications:
-
Deglycosylation : In vivo, glycosidases may cleave the gentiobioside side chains, releasing the aglycone (toralactone) .
-
Oxidation : The naphthopyrone core may undergo hepatic cytochrome P450-mediated oxidation, forming quinone derivatives .
Key Research Findings
-
Stereochemical Outcomes : Glycosylation proceeds with retention of β-configuration due to neighboring group participation .
-
Synthetic Challenges : The tetraglucosyl chain introduces steric hindrance, necessitating iterative protection/deprotection strategies .
Comparative Reactivity with Analogues
Unresolved Questions
Scientific Research Applications
Anti-Inflammatory Properties
Mechanism of Action:
Cassiaside C has been shown to inhibit the M1 polarization of macrophages, a critical process in the inflammatory response. By downregulating glycolysis, this compound reduces the expression of pro-inflammatory cytokines such as TNF-α, IL-6, and MCP-1 in macrophages stimulated by lipopolysaccharides (LPS) and interferon-gamma (IFN-γ) . This modulation of macrophage polarization could be a promising therapeutic strategy for chronic inflammatory diseases.
Key Findings:
- Inhibition of Glycolysis: this compound decreases lactate production and enhances oxidative phosphorylation in M1 polarized macrophages .
- Cytokine Suppression: The compound significantly lowers the levels of inducible nitric oxide synthase and cyclooxygenase-2, which are upregulated during inflammation .
Neuroprotective Effects
This compound exhibits potential neuroprotective effects, particularly relevant in the context of neurodegenerative diseases such as Alzheimer's disease (AD). Research indicates that it can inhibit beta-secretase 1 (BACE1), an enzyme involved in the formation of amyloid-beta plaques, a hallmark of AD .
Studies Highlighting Neuroprotection:
- BACE1 Inhibition: this compound shows mixed-type inhibition against BACE1, suggesting its utility in reducing amyloid plaque formation .
- Cell Viability Improvement: In models of neuronal toxicity induced by amyloid-beta oligomers, this compound enhances cell survival and function .
Antiallergic Activity
This compound has demonstrated significant antiallergic properties by inhibiting histamine release from mast cells during antigen-antibody reactions. This activity positions it as a candidate for developing treatments for allergic conditions .
Antioxidant Properties
The compound's antioxidant capacity contributes to its overall pharmacological profile. It helps mitigate oxidative stress, which is implicated in various chronic diseases .
Potential Applications in Cancer Therapy
Emerging studies suggest that this compound may possess anticancer properties by modulating cellular pathways associated with tumor growth and metastasis. Its ability to inhibit certain signaling pathways could be leveraged in cancer treatment strategies .
Summary Table of Applications
Mechanism of Action
Cassiaside C exerts its effects by modulating various molecular targets and pathways:
Anti-inflammatory Action: this compound inhibits the polarization of M1 macrophages by downregulating glycolysis.
Antioxidant Action: The compound scavenges free radicals and reduces oxidative stress, protecting cells from damage.
Molecular Pathways: this compound affects the phosphoinositide 3-kinases (PI3K)/AKT/mammalian target of rapamycin complex 1 (mTORC1) signaling pathway, leading to reduced glycolysis and lactate production.
Comparison with Similar Compounds
Cassiaside C belongs to a family of naphthopyrone glycosides. Structurally and functionally related compounds include Cassiaside B2 , Cassiaside C2 , and Aurantio-Obtusin . Below is a detailed comparison:
Table 1: Structural and Functional Comparison of this compound with Analogues
Structural Similarities and Differences
- Sugar Moieties : this compound contains a gentiobioside group (two glucose units), whereas Cassiaside B2 and C2 likely differ in glycosylation patterns, altering solubility and bioavailability .
- Core Structure: Aurantio-Obtusin, an anthraquinone, lacks the glycoside chain but shares a naphthopyrone backbone, enabling NF-κB inhibition .
Functional Divergence
- This compound vs. Cassiaside B2: While both are glycosides from Cassiae semen, Cassiaside B2 targets phosphatases and monoamine oxidases, suggesting a role in metabolic and neurological disorders, unlike this compound’s focus on inflammation and glycolysis .
- This compound vs. Aurantio-Obtusin : The absence of a glycoside group in Aurantio-Obtusin limits its solubility but enhances membrane permeability, favoring direct NF-κB pathway modulation .
Pharmacokinetic Considerations
Biological Activity
Cassiaside C, a phenolic glycoside derived from Cassia obtusifolia, has garnered attention for its diverse biological activities, including antioxidant, anti-inflammatory, and antimicrobial properties. This article synthesizes current research findings on the biological activity of this compound, supported by data tables and case studies.
Chemical Structure and Properties
This compound is characterized by its complex structure, which includes multiple sugar moieties linked to a phenolic backbone. This structural composition contributes to its bioactivity. The compound's molecular formula is typically represented as .
1. Antioxidant Activity
This compound exhibits significant antioxidant properties, which are crucial in mitigating oxidative stress and preventing cellular damage. Research indicates that it can scavenge free radicals effectively, contributing to its potential therapeutic applications in oxidative stress-related diseases.
Table 1: Antioxidant Activity of this compound
Study | Method Used | IC50 (µg/mL) | Findings |
---|---|---|---|
DPPH Assay | 15.4 | Effective radical scavenging activity observed. | |
ABTS Assay | 12.3 | Higher antioxidant capacity compared to standard antioxidants. |
2. Anti-Inflammatory Effects
The anti-inflammatory properties of this compound have been demonstrated in various studies. It inhibits the production of pro-inflammatory cytokines and enzymes involved in inflammatory pathways.
Table 2: Anti-Inflammatory Activity of this compound
Study | Model Used | Concentration (µg/mL) | Inhibition (%) |
---|---|---|---|
RAW264.7 Macrophages | 25 | 70% inhibition of NO production | |
Murine Model (LPS-induced) | 50 | Reduced IL-1β secretion significantly |
3. Antimicrobial Activity
This compound has shown promising antimicrobial effects against various bacterial strains, including methicillin-resistant Staphylococcus aureus (MRSA). This activity is attributed to its ability to disrupt bacterial cell membranes and inhibit growth.
Table 3: Antimicrobial Activity of this compound
Case Study 1: Antioxidant Application in Food Preservation
A study evaluated the use of this compound as a natural preservative in food products. The addition of this compound significantly reduced lipid peroxidation in meat products, extending shelf life while maintaining quality.
Case Study 2: Anti-inflammatory Treatment in Animal Models
In a controlled experiment involving mice with induced inflammation, treatment with this compound resulted in decreased paw edema and lower levels of inflammatory markers compared to control groups. This suggests its potential as an anti-inflammatory agent in clinical settings.
Q & A
Basic Research Questions
Q. What validated methodologies are recommended for isolating and purifying Cassiaside C from plant sources?
- Methodological Answer : Isolation typically involves solvent extraction (e.g., ethanol or methanol) followed by chromatographic techniques such as column chromatography (silica gel or Sephadex LH-20) and HPLC for purification. Key parameters include solvent polarity gradients and detection wavelengths (e.g., 254 nm for UV-vis) . Purity validation requires NMR (¹H, ¹³C) and mass spectrometry (LC-MS/MS), with thresholds ≥95% purity for pharmacological studies. Reproducibility hinges on documenting solvent ratios, temperature, and column pressure in experimental protocols .
Q. How do researchers standardize bioactivity assays for this compound’s antioxidant properties?
- Answer : Standardized assays include DPPH radical scavenging, FRAP, and ORAC, with strict controls for pH, temperature, and reaction time. For example, DPPH assays require dissolving this compound in DMSO (≤0.1% final concentration to avoid solvent interference) and comparing against Trolox or ascorbic acid standards . Data normalization to mg/g dry weight or IC₅₀ values ensures cross-study comparability. Include positive/negative controls and triplicate measurements to minimize batch variability .
Q. What in vitro models are most suitable for preliminary toxicity screening of this compound?
- Answer : Common models include:
- Cell lines : HepG2 (hepatotoxicity), HEK293 (renal), and Caco-2 (intestinal permeability).
- Assays : MTT or resazurin for cell viability, complemented by LDH release for membrane integrity. Dose ranges should span 1–100 μM, with exposure times ≤72 hours . Pre-test solubility in culture media (e.g., PBS with 0.1% DMSO) is critical to avoid false positives .
Advanced Research Questions
Q. How can researchers resolve contradictions in reported antioxidant vs. pro-oxidant effects of this compound?
- Answer : Contradictions often arise from assay conditions (e.g., ROS concentration, cell type). For example, pro-oxidant effects may dominate in cancer cells (e.g., A549) under high-dose exposure (≥50 μM) due to Fenton-like reactions. Advanced approaches:
- Dual ROS probes : Use CellROX (general ROS) and MitoSOX (mitochondrial-specific) to localize effects.
- Transcriptomic analysis : RNA-seq to identify Nrf2/Keap1 pathway modulation vs. pro-apoptotic Bax/Bcl-2 shifts .
- Meta-analysis : Systematically compare studies using PRISMA guidelines, stratifying by dose, model, and assay type .
Q. What experimental designs are optimal for elucidating this compound’s mechanism of action in chronic inflammation?
- Answer : A tiered approach is recommended:
In vitro : LPS-stimulated RAW264.7 macrophages with ELISA for TNF-α, IL-6, and NF-κB nuclear translocation (immunofluorescence).
In vivo : Collagen-induced arthritis (CIA) in mice, administering 10–50 mg/kg this compound orally for 21 days. Measure joint swelling, serum cytokines, and histopathology .
Omics integration : Proteomics (2D-DIGE) and metabolomics (LC-QTOF-MS) to map pathway interactions (e.g., COX-2 inhibition vs. AMPK activation) .
Q. How should researchers address batch-to-batch variability in this compound’s pharmacokinetic (PK) data?
- Answer : Variability stems from differences in extraction methods, purity, and animal models. Mitigation strategies:
- Standardized PK protocols : Administer via IV (1 mg/kg) and oral (10 mg/kg) routes in Sprague-Dawley rats, with serial plasma sampling over 24h. Use a validated LC-MS/MS method (LLOQ ≤1 ng/mL) .
- Population PK modeling : Non-linear mixed-effects modeling (e.g., Monolix) to account for inter-individual variability .
- Cross-lab validation : Share reference samples between labs to calibrate analytical instruments .
Q. Data Synthesis and Contradiction Management
Q. What statistical methods are appropriate for analyzing dose-response inconsistencies in this compound’s anticancer studies?
- Answer :
- Hill slope analysis : Compare sigmoidal curves across cell lines (e.g., IC₅₀ in MCF-7 vs. HeLa).
- ANCOVA : Adjust for covariates like doubling time and seeding density .
- Bayesian meta-regression : Pool data from heterogeneous studies, weighting by sample size and assay precision .
Q. How can researchers integrate fragmented data on this compound’s synergistic effects with chemotherapeutics?
- Answer :
- Isobologram analysis : Calculate combination indices (CI) for this compound + doxorubicin/paclitaxel. CI <1 indicates synergy .
- Transcriptome clustering : Use hierarchical clustering (GenePattern) to identify overlapping gene targets (e.g., TOP2A, BCL2) .
- Table : Synergy Data Summary
Combination Partner | Cell Line | CI Value | Key Pathways |
---|---|---|---|
Doxorubicin | MCF-7 | 0.45 | DNA repair, apoptosis |
Paclitaxel | A549 | 0.72 | Mitotic arrest |
Q. Ethical and Reproducibility Considerations
Q. What guidelines ensure ethical rigor in this compound’s in vivo studies?
- Answer :
- ARRIVE 2.0 : Full disclosure of animal numbers, randomization, and blinding.
- 3Rs compliance : Replace rodent models with zebrafish embryos (FET assay) where feasible .
- Data transparency : Deposit raw data (e.g., RNA-seq files) in public repositories like GEO or PRIDE .
Q. How can researchers enhance reproducibility in this compound studies?
- Answer :
- MIAME compliance : Document all experimental conditions (e.g., exact HPLC gradients).
- Negative result reporting : Publish null findings (e.g., lack of cytotoxicity in normal cells) to reduce publication bias .
- Collaborative verification : Cross-validate findings via multi-center trials, using harmonized protocols .
Properties
IUPAC Name |
10-hydroxy-7-methoxy-3-methyl-9-[3,4,5-trihydroxy-6-[[3,4,5-trihydroxy-6-(hydroxymethyl)oxan-2-yl]oxymethyl]oxan-2-yl]oxybenzo[g]isochromen-1-one | |
---|---|---|
Details | Computed by LexiChem 2.6.6 (PubChem release 2019.06.18) | |
Source | PubChem | |
URL | https://pubchem.ncbi.nlm.nih.gov | |
Description | Data deposited in or computed by PubChem | |
InChI |
InChI=1S/C27H32O15/c1-9-3-10-4-11-5-12(37-2)6-13(16(11)20(31)17(10)25(36)39-9)40-27-24(35)22(33)19(30)15(42-27)8-38-26-23(34)21(32)18(29)14(7-28)41-26/h3-6,14-15,18-19,21-24,26-35H,7-8H2,1-2H3 | |
Details | Computed by InChI 1.0.5 (PubChem release 2019.06.18) | |
Source | PubChem | |
URL | https://pubchem.ncbi.nlm.nih.gov | |
Description | Data deposited in or computed by PubChem | |
InChI Key |
GBGJNKYTLIUCMX-UHFFFAOYSA-N | |
Details | Computed by InChI 1.0.5 (PubChem release 2019.06.18) | |
Source | PubChem | |
URL | https://pubchem.ncbi.nlm.nih.gov | |
Description | Data deposited in or computed by PubChem | |
Canonical SMILES |
CC1=CC2=CC3=CC(=CC(=C3C(=C2C(=O)O1)O)OC4C(C(C(C(O4)COC5C(C(C(C(O5)CO)O)O)O)O)O)O)OC | |
Details | Computed by OEChem 2.1.5 (PubChem release 2019.06.18) | |
Source | PubChem | |
URL | https://pubchem.ncbi.nlm.nih.gov | |
Description | Data deposited in or computed by PubChem | |
Molecular Formula |
C27H32O15 | |
Details | Computed by PubChem 2.1 (PubChem release 2019.06.18) | |
Source | PubChem | |
URL | https://pubchem.ncbi.nlm.nih.gov | |
Description | Data deposited in or computed by PubChem | |
Molecular Weight |
596.5 g/mol | |
Details | Computed by PubChem 2.1 (PubChem release 2021.05.07) | |
Source | PubChem | |
URL | https://pubchem.ncbi.nlm.nih.gov | |
Description | Data deposited in or computed by PubChem | |
Physical Description |
Solid | |
Record name | Cassiaside C | |
Source | Human Metabolome Database (HMDB) | |
URL | http://www.hmdb.ca/metabolites/HMDB0038550 | |
Description | The Human Metabolome Database (HMDB) is a freely available electronic database containing detailed information about small molecule metabolites found in the human body. | |
Explanation | HMDB is offered to the public as a freely available resource. Use and re-distribution of the data, in whole or in part, for commercial purposes requires explicit permission of the authors and explicit acknowledgment of the source material (HMDB) and the original publication (see the HMDB citing page). We ask that users who download significant portions of the database cite the HMDB paper in any resulting publications. | |
CAS No. |
119170-52-4 | |
Record name | Cassiaside C | |
Source | Human Metabolome Database (HMDB) | |
URL | http://www.hmdb.ca/metabolites/HMDB0038550 | |
Description | The Human Metabolome Database (HMDB) is a freely available electronic database containing detailed information about small molecule metabolites found in the human body. | |
Explanation | HMDB is offered to the public as a freely available resource. Use and re-distribution of the data, in whole or in part, for commercial purposes requires explicit permission of the authors and explicit acknowledgment of the source material (HMDB) and the original publication (see the HMDB citing page). We ask that users who download significant portions of the database cite the HMDB paper in any resulting publications. | |
Melting Point |
235 - 237 °C | |
Record name | Cassiaside C | |
Source | Human Metabolome Database (HMDB) | |
URL | http://www.hmdb.ca/metabolites/HMDB0038550 | |
Description | The Human Metabolome Database (HMDB) is a freely available electronic database containing detailed information about small molecule metabolites found in the human body. | |
Explanation | HMDB is offered to the public as a freely available resource. Use and re-distribution of the data, in whole or in part, for commercial purposes requires explicit permission of the authors and explicit acknowledgment of the source material (HMDB) and the original publication (see the HMDB citing page). We ask that users who download significant portions of the database cite the HMDB paper in any resulting publications. | |
Retrosynthesis Analysis
AI-Powered Synthesis Planning: Our tool employs the Template_relevance Pistachio, Template_relevance Bkms_metabolic, Template_relevance Pistachio_ringbreaker, Template_relevance Reaxys, Template_relevance Reaxys_biocatalysis model, leveraging a vast database of chemical reactions to predict feasible synthetic routes.
One-Step Synthesis Focus: Specifically designed for one-step synthesis, it provides concise and direct routes for your target compounds, streamlining the synthesis process.
Accurate Predictions: Utilizing the extensive PISTACHIO, BKMS_METABOLIC, PISTACHIO_RINGBREAKER, REAXYS, REAXYS_BIOCATALYSIS database, our tool offers high-accuracy predictions, reflecting the latest in chemical research and data.
Strategy Settings
Precursor scoring | Relevance Heuristic |
---|---|
Min. plausibility | 0.01 |
Model | Template_relevance |
Template Set | Pistachio/Bkms_metabolic/Pistachio_ringbreaker/Reaxys/Reaxys_biocatalysis |
Top-N result to add to graph | 6 |
Feasible Synthetic Routes
Disclaimer and Information on In-Vitro Research Products
Please be aware that all articles and product information presented on BenchChem are intended solely for informational purposes. The products available for purchase on BenchChem are specifically designed for in-vitro studies, which are conducted outside of living organisms. In-vitro studies, derived from the Latin term "in glass," involve experiments performed in controlled laboratory settings using cells or tissues. It is important to note that these products are not categorized as medicines or drugs, and they have not received approval from the FDA for the prevention, treatment, or cure of any medical condition, ailment, or disease. We must emphasize that any form of bodily introduction of these products into humans or animals is strictly prohibited by law. It is essential to adhere to these guidelines to ensure compliance with legal and ethical standards in research and experimentation.