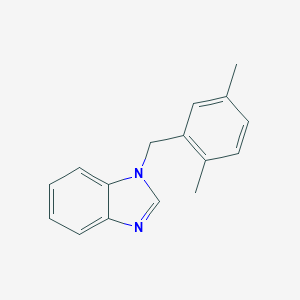
1-(2,5-dimethylbenzyl)-1H-benzimidazole
Overview
Description
1-(2,5-Dimethylbenzyl)-1H-benzimidazole is a substituted benzimidazole derivative characterized by a benzyl group substituted with methyl groups at the 2- and 5-positions of the benzene ring, attached to the N1 position of the benzimidazole core. The benzimidazole scaffold itself is a bicyclic aromatic heterocycle formed by fusing a benzene ring with an imidazole ring, conferring both rigidity and diverse electronic properties . This compound’s structural uniqueness lies in its 2,5-dimethylbenzyl substituent, which enhances lipophilicity and may influence binding interactions in biological systems.
Preparation Methods
Classical Alkylation of Benzimidazole with 2,5-Dimethylbenzyl Chloride
The most direct route to 1-(2,5-dimethylbenzyl)-1H-benzimidazole involves the N-alkylation of benzimidazole using 2,5-dimethylbenzyl chloride under basic conditions. This method leverages the nucleophilic substitution mechanism, where the deprotonated nitrogen of benzimidazole attacks the electrophilic benzyl chloride derivative.
In a representative procedure, benzimidazole (1.0 equiv) is dissolved in a polar aprotic solvent such as dimethylformamide (DMF) or dimethyl sulfoxide (DMSO). Potassium hydroxide (1.2 equiv) is added to deprotonate the benzimidazole, followed by dropwise addition of 2,5-dimethylbenzyl chloride (1.1 equiv) at 0–5°C to minimize side reactions . The mixture is stirred at room temperature for 12–24 hours, after which the product is isolated via aqueous workup and recrystallization from ethanol/water. This method typically achieves yields of 70–85%, with purity exceeding 95% as confirmed by HPLC .
Key Variables:
-
Solvent Selection: DMSO enhances reaction rates due to its high polarity but complicates purification. Ethanol or methanol alternatives reduce side products but require longer reaction times.
-
Base Optimization: KOH outperforms NaOH or NaH in minimizing O-alkylation byproducts.
-
Temperature Control: Reactions conducted above 25°C risk dimerization of 2,5-dimethylbenzyl chloride.
Microwave-Assisted Synthesis via 4-Methyl-o-Phenylenediamine Condensation
An alternative approach constructs the benzimidazole core in situ before introducing the 2,5-dimethylbenzyl group. 4-Methyl-o-phenylenediamine serves as the starting material, condensed with formic acid or its derivatives under microwave irradiation to form the benzimidazole intermediate .
In a optimized protocol, 4-methyl-o-phenylenediamine (1.0 equiv) and formic acid (2.0 equiv) are mixed in ethanol with ammonium chloride (0.5 equiv) as a catalyst. The reaction is irradiated in a microwave reactor at 400 W for 4–8 minutes, achieving cyclization to 5,6-dimethyl-1H-benzimidazole . Subsequent N-alkylation with 2,5-dimethylbenzyl bromide under phase-transfer conditions (tetrabutylammonium bromide, 50°C, 6 hours) furnishes the target compound in 65–78% overall yield.
Advantages of Microwave Synthesis:
-
Time Efficiency: Reduces reaction time from hours to minutes.
-
Improved Selectivity: Higher regiocontrol in benzimidazole formation compared to thermal methods.
Acid-Catalyzed Cyclocondensation Strategies
Phosphorus pentoxide (P₂O₅) in methanesulfonic acid (MSA) provides a robust medium for one-pot syntheses combining cyclization and alkylation steps. This method is adapted from patented protocols for related benzimidazole derivatives .
A typical procedure involves dissolving 2,5-dimethylbenzyl alcohol (1.0 equiv) and o-phenylenediamine (1.0 equiv) in MSA at 120°C. P₂O₅ (1.5 equiv) is added portionwise to catalyze dehydration, promoting simultaneous benzimidazole ring formation and N-alkylation. After 3 hours at 130–145°C, the mixture is quenched with ice water, neutralized with NaOH, and extracted with isopropanol. This route achieves 60–72% yield but requires careful temperature control to avoid charring .
Mechanistic Insights:
-
P₂O₅ acts as a dual dehydrating agent and acid catalyst, facilitating both imine formation and electrophilic alkylation.
-
MSA stabilizes reactive intermediates, reducing side reactions such as polymerization.
Transition Metal-Catalyzed Dehydrogenative Coupling
Emerging methodologies employ transition metal complexes to enable dehydrogenative coupling between 2,5-dimethylbenzyl alcohol and benzimidazole precursors. A cobalt-pincer complex (e.g., (PNN)Co(CO)) catalyzes the reaction at 150°C under inert atmosphere, converting primary alcohols directly to alkylating agents via hydrogen transfer .
In this system, o-phenylenediamine (1.0 equiv) and 2,5-dimethylbenzyl alcohol (1.2 equiv) react in toluene with 2 mol% catalyst. The process generates water as the sole byproduct, achieving 55–68% yield after 24 hours. While less efficient than classical alkylation, this method offers atom economy and avoids halogenated reagents .
Comparative Analysis of Synthetic Methodologies
Critical Observations:
-
Classical alkylation remains the most reliable for scale-up due to predictable yields and straightforward purification.
-
Microwave methods excel in rapid synthesis but face limitations in solvent compatibility and equipment availability.
-
Transition metal catalysis, while environmentally favorable, requires further optimization to rival traditional approaches.
Chemical Reactions Analysis
Types of Reactions: 1-(2,5-Dimethylbenzyl)-1H-benzimidazole can undergo various chemical reactions, including:
Oxidation: The compound can be oxidized using oxidizing agents such as hydrogen peroxide or potassium permanganate, leading to the formation of benzimidazole derivatives with different functional groups.
Reduction: Reduction reactions can be carried out using reducing agents like sodium borohydride or lithium aluminum hydride, which can modify the benzimidazole ring or the substituent groups.
Common Reagents and Conditions:
Oxidation: Hydrogen peroxide, potassium permanganate, and other oxidizing agents under acidic or basic conditions.
Reduction: Sodium borohydride, lithium aluminum hydride, and other reducing agents under anhydrous conditions.
Substitution: Electrophiles or nucleophiles in the presence of catalysts such as Lewis acids or bases.
Major Products: The major products formed from these reactions depend on the specific reagents and conditions used. For example, oxidation may yield benzimidazole derivatives with hydroxyl or carbonyl groups, while reduction can produce amine or alkyl derivatives.
Scientific Research Applications
Antiproliferative Activity
Research indicates that 1-(2,5-dimethylbenzyl)-1H-benzimidazole and its derivatives exhibit notable antiproliferative effects against various cancer cell lines. A study highlighted that certain derivatives demonstrated significant inhibition against the MDA-MB-231 breast cancer cell line with IC50 values ranging from 16.38 μM to over 100 μM depending on the specific substitution patterns on the benzimidazole ring .
Key Findings:
- Compound 2g showed the best activity with an IC50 of 16.38 μM.
- The presence of alkyl substitutions on the benzimidazole ring enhanced cytotoxicity due to increased lipophilicity, facilitating better membrane penetration .
Antifungal Activity
The antifungal potential of this compound has been evaluated against common fungal strains such as Candida albicans and Aspergillus niger. The minimum inhibitory concentration (MIC) values for some derivatives were reported at 64 μg/mL for both strains, indicating moderate antifungal activity .
Antibacterial Activity
The antibacterial efficacy of this compound has also been investigated. It has shown promising results against various bacterial strains, including methicillin-resistant Staphylococcus aureus (MRSA). The MIC values were significantly lower than those of standard antibiotics like amikacin, suggesting that these compounds could be developed as alternative antibacterial agents .
Table: Antibacterial Activity of Selected Derivatives
Compound | Bacterial Strain | MIC (μg/mL) |
---|---|---|
2g | Streptococcus faecalis | 8 |
2g | Staphylococcus aureus | 4 |
2g | MRSA | 4 |
Case Studies and Research Findings
Several studies have documented the synthesis and evaluation of benzimidazole derivatives including this compound. For instance:
- A series of N-alkylated derivatives were synthesized and characterized using techniques such as NMR and FTIR spectroscopy. These derivatives were screened for their biological activities, revealing a correlation between structural modifications and enhanced pharmacological effects .
- Another study focused on the anti-cancer activity of a new class of benzimidazole derivatives, demonstrating their ability to induce apoptosis in cancer cells through mitochondrial pathways .
Mechanism of Action
The mechanism of action of 1-(2,5-dimethylbenzyl)-1H-benzimidazole involves its interaction with molecular targets such as enzymes, receptors, and nucleic acids. The compound can inhibit the activity of certain enzymes by binding to their active sites, thereby blocking substrate access. Additionally, it can interact with receptors on cell surfaces, modulating signal transduction pathways and affecting cellular functions.
Comparison with Similar Compounds
Comparative Analysis with Structurally Similar Benzimidazole Derivatives
Substituent Position and Bioactivity
The position and nature of substituents on the benzimidazole core critically determine biological activity. Below is a comparison with key analogs:
Key Observations :
- Halogen vs. Methyl Substituents : Halogenated derivatives (e.g., 4c ) exhibit enhanced antitumor activity compared to methyl-substituted analogs, likely due to increased electrophilicity and stronger interactions with cellular targets.
- N1-Substitution : The N1-(2,5-dimethylbenzyl) group in the target compound may improve pharmacokinetic properties (e.g., metabolic stability) over smaller substituents like methyl or phenyl .
- Sulfinyl vs. Benzyl Groups: Omeprazole’s sulfinyl group enables covalent binding to proton pumps, a mechanism distinct from the non-covalent interactions expected for the dimethylbenzyl analog .
Pharmacological and Physicochemical Properties
- Enzyme Inhibition : While the dimethylbenzyl derivative’s enzyme inhibition profile is undocumented, structurally related N-phenyl derivatives show Sirtuin inhibitory activity, suggesting a possible target class .
- Antimicrobial Activity : Bis-benzimidazoles with chloromethyl substituents (e.g., 1a-c ) exhibit broad-spectrum antibacterial effects, but methyl groups may reduce this activity due to decreased electrophilicity .
Biological Activity
1-(2,5-Dimethylbenzyl)-1H-benzimidazole is a derivative of benzimidazole, a class of compounds known for their diverse biological activities. This article explores the biological activity of this specific compound, focusing on its pharmacological effects, mechanisms of action, and potential therapeutic applications. The information is drawn from various studies and reviews that highlight the compound's significance in medicinal chemistry.
Chemical Structure and Properties
The compound this compound features a benzimidazole core substituted with a 2,5-dimethylbenzyl group. This substitution can influence its lipophilicity and biological activity, making it a subject of interest in drug development.
Antiproliferative Activity
Research indicates that benzimidazole derivatives exhibit significant antiproliferative effects against various cancer cell lines. For instance, studies have shown that certain derivatives demonstrate IC50 values (the concentration required to inhibit cell growth by 50%) in the low micromolar range. Specifically, compounds with alkyl substitutions at the N-1 position have been noted to enhance anticancer efficacy:
Compound | Cell Line | IC50 (μM) |
---|---|---|
2g | MDA-MB-231 | 16.38 |
2d | MDA-MB-231 | 29.39 |
1a | MDA-MB-231 | >100 |
These results suggest that structural modifications can significantly impact the antiproliferative activity of benzimidazole derivatives .
Antibacterial and Antifungal Activity
The antibacterial and antifungal properties of benzimidazole derivatives have been widely studied. The minimal inhibitory concentrations (MIC) for various strains have been measured, demonstrating notable efficacy:
Pathogen | MIC (μg/mL) |
---|---|
Staphylococcus aureus | 4 |
Streptococcus faecalis | 8 |
Candida albicans | 64 |
These findings indicate that compounds like this compound could serve as potential agents against resistant bacterial strains and fungal infections .
Benzimidazole derivatives may exert their biological effects through several mechanisms:
- Topoisomerase Inhibition : Some studies have shown that benzimidazoles can inhibit DNA topoisomerases, enzymes crucial for DNA replication and transcription .
- Microtubule Disruption : Similar to other known antitumor agents, these compounds may interfere with microtubule dynamics, leading to apoptosis in cancer cells .
- Enzyme Inhibition : Compounds have also been identified as inhibitors of dihydrofolate reductase (DHFR), an enzyme involved in folate metabolism crucial for DNA synthesis .
Case Studies
Several case studies illustrate the therapeutic potential of benzimidazole derivatives:
- Anticancer Activity : A study evaluated a series of substituted benzimidazoles against breast cancer cell lines, revealing that modifications at the N-1 position significantly enhanced cytotoxicity.
- Antimicrobial Efficacy : A comparative analysis of various benzimidazole derivatives highlighted their effectiveness against both Gram-positive and Gram-negative bacteria, with some derivatives outperforming standard antibiotics .
Q & A
Basic Research Questions
Q. What are the standard synthetic protocols for 1-(2,5-dimethylbenzyl)-1H-benzimidazole, and how are intermediates characterized?
The synthesis typically involves multi-step reactions starting with o-phenylenediamine derivatives. For example:
- Step 1 : Condensation of o-phenylenediamine with carbon disulfide in ethanol under alkaline conditions to form 1H-benzo[d]imidazole-2-thiol .
- Step 2 : Reaction with hydrazine hydrate to yield 2-hydrazinyl-1H-benzo[d]imidazole, confirmed by shifts in IR bands (e.g., loss of S-H at 2634 cm⁻¹ and appearance of N-H stretches at 3464 cm⁻¹) .
- Step 3 : Alkylation with 2,5-dimethylbenzyl chloride to introduce the dimethylbenzyl group.
Characterization relies on elemental analysis , IR , ¹H/¹³C-NMR , and mass spectrometry . For instance, NMR peaks at δ12.31 (S-H) and δ10.93 (N-H) confirm intermediate structures .
Q. How do substituents on the benzimidazole core influence spectroscopic properties?
Substituents alter electronic environments, detectable via:
- IR : Shifts in N-H (3395–3464 cm⁻¹) and aromatic C-H (3024–3100 cm⁻¹) stretches .
- NMR : Aromatic proton signals (δ6.5–8.5 ppm) and carbon shifts (e.g., δ151.93 for N=C-N in benzimidazole) .
- Mass spectrometry : Molecular ion peaks (e.g., m/z 263 for C₁₆H₁₇N₂ derivatives) .
Advanced Research Questions
Q. How can structure-activity relationship (SAR) studies optimize antifungal activity in this compound derivatives?
Key SAR insights include:
- Substituent position : 2,5-dimethylbenzyl groups enhance lipophilicity, improving membrane penetration in fungal cells .
- Electron-withdrawing groups : Nitro or halogen substituents at the benzyl moiety increase antifungal efficacy by modulating redox potentials .
- Hybrid derivatives : Coupling with hydrazine carboxamides (e.g., compound 4a-t ) improves binding to fungal cytochrome P450 enzymes .
Validation requires in vitro MIC assays against Candida spp. and molecular docking to Lanosterol 14α-demethylase .
Q. What computational strategies resolve contradictions in biological data across benzimidazole derivatives?
Discrepancies in activity (e.g., varying IC₅₀ values) arise from:
- Solubility differences : Use QSAR models with logP and polar surface area descriptors to predict bioavailability .
- Assay variability : Standardize protocols (e.g., broth microdilution for antifungal tests) and apply 3D-QSAR to align steric/electronic features with activity .
- Metabolic stability : ADME predictions (e.g., SwissADME) identify derivatives prone to rapid hepatic clearance .
Q. How can microwave-assisted synthesis improve yield and purity of benzimidazole derivatives?
Microwave irradiation accelerates reaction kinetics via uniform heating:
- Example : Pd(OAc)₂-catalyzed Heck/Suzuki couplings with silyl-substituted benzimidazoles achieve >85% yield in 30 minutes vs. 12 hours conventionally .
- Benefits : Reduced side reactions (e.g., oxidation), confirmed by HPLC purity >98% .
Q. What mechanistic approaches validate the anti-inflammatory activity of this compound derivatives?
- In vitro : COX-2 inhibition assays (IC₅₀ <10 µM for derivatives like A2 and A8 ) .
- In vivo : Carrageenan-induced rat paw edema models, with dose-dependent reduction in TNF-α and IL-6 levels .
- Molecular dynamics : Simulations reveal stable binding to COX-2’s arachidonic acid pocket via hydrophobic interactions .
Q. How can formulation strategies enhance topical delivery of benzimidazole derivatives for burn treatment?
- Cubosome hydrogels : Load 1-benzyl-1H-benzimidazole into glyceryl monooleate nanoparticles, achieving sustained release (>72 hours) .
- Characterization : Dynamic light scattering (PDI <0.2) and TEM confirm cubosome morphology (cubic phase, 100–200 nm) .
- Efficacy testing : In vivo burn models show accelerated re-epithelialization and reduced bacterial load vs. controls .
Q. Methodological Considerations
Q. What analytical techniques resolve structural ambiguities in benzimidazole derivatives?
- X-ray crystallography : Determines absolute configuration (e.g., compound 7 in silyl-substituted derivatives) .
- 2D NMR : COSY and HMQC distinguish overlapping aromatic protons .
- High-resolution mass spectrometry (HRMS) : Confirms molecular formulas (e.g., [M+H]⁺ at m/z 281.1542 for C₁₇H₁₇N₂O) .
Q. How do solvent-free synthesis and organocatalysis improve sustainability in benzimidazole chemistry?
Properties
IUPAC Name |
1-[(2,5-dimethylphenyl)methyl]benzimidazole | |
---|---|---|
Source | PubChem | |
URL | https://pubchem.ncbi.nlm.nih.gov | |
Description | Data deposited in or computed by PubChem | |
InChI |
InChI=1S/C16H16N2/c1-12-7-8-13(2)14(9-12)10-18-11-17-15-5-3-4-6-16(15)18/h3-9,11H,10H2,1-2H3 | |
Source | PubChem | |
URL | https://pubchem.ncbi.nlm.nih.gov | |
Description | Data deposited in or computed by PubChem | |
InChI Key |
KAUYGLBHEVVUBY-UHFFFAOYSA-N | |
Source | PubChem | |
URL | https://pubchem.ncbi.nlm.nih.gov | |
Description | Data deposited in or computed by PubChem | |
Canonical SMILES |
CC1=CC(=C(C=C1)C)CN2C=NC3=CC=CC=C32 | |
Source | PubChem | |
URL | https://pubchem.ncbi.nlm.nih.gov | |
Description | Data deposited in or computed by PubChem | |
Molecular Formula |
C16H16N2 | |
Source | PubChem | |
URL | https://pubchem.ncbi.nlm.nih.gov | |
Description | Data deposited in or computed by PubChem | |
Molecular Weight |
236.31 g/mol | |
Source | PubChem | |
URL | https://pubchem.ncbi.nlm.nih.gov | |
Description | Data deposited in or computed by PubChem | |
Disclaimer and Information on In-Vitro Research Products
Please be aware that all articles and product information presented on BenchChem are intended solely for informational purposes. The products available for purchase on BenchChem are specifically designed for in-vitro studies, which are conducted outside of living organisms. In-vitro studies, derived from the Latin term "in glass," involve experiments performed in controlled laboratory settings using cells or tissues. It is important to note that these products are not categorized as medicines or drugs, and they have not received approval from the FDA for the prevention, treatment, or cure of any medical condition, ailment, or disease. We must emphasize that any form of bodily introduction of these products into humans or animals is strictly prohibited by law. It is essential to adhere to these guidelines to ensure compliance with legal and ethical standards in research and experimentation.