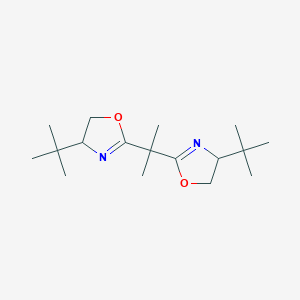
2,2'-(2,2-propanediyl)bis(4-tert-butyl-4,5-dihydro-1,3-oxazole)
Overview
Description
“2,2’-(2,2-propanediyl)bis(4-tert-butyl-4,5-dihydro-1,3-oxazole)” is a chemical compound with the molecular formula C17H30N2O2 . It is a solid substance at room temperature . The compound has a molecular weight of 294.44 .
Molecular Structure Analysis
The molecular structure of this compound consists of a propane-2,2-diyl group (a three-carbon chain with two substituents on the central carbon) linked to two 4,5-dihydro-1,3-oxazole rings, each of which is substituted with a tert-butyl group . The InChI code for this compound is 1S/C17H30N2O2/c1-15(2,3)11-9-20-13(18-11)17(7,8)14-19-12(10-21-14)16(4,5)6/h11-12H,9-10H2,1-8H3/t11-,12-/m0/s1 .Physical and Chemical Properties Analysis
This compound is a solid at room temperature . It has a molecular weight of 294.44 .Safety and Hazards
The compound is labeled with the GHS07 pictogram, indicating that it can cause skin irritation, serious eye irritation, and may cause respiratory irritation . The precautionary statements associated with this compound are P305+P351+P338, which advise to rinse cautiously with water for several minutes in case of contact with eyes .
Properties
IUPAC Name |
4-tert-butyl-2-[2-(4-tert-butyl-4,5-dihydro-1,3-oxazol-2-yl)propan-2-yl]-4,5-dihydro-1,3-oxazole | |
---|---|---|
Details | Computed by LexiChem 2.6.6 (PubChem release 2019.06.18) | |
Source | PubChem | |
URL | https://pubchem.ncbi.nlm.nih.gov | |
Description | Data deposited in or computed by PubChem | |
InChI |
InChI=1S/C17H30N2O2/c1-15(2,3)11-9-20-13(18-11)17(7,8)14-19-12(10-21-14)16(4,5)6/h11-12H,9-10H2,1-8H3 | |
Details | Computed by InChI 1.0.5 (PubChem release 2019.06.18) | |
Source | PubChem | |
URL | https://pubchem.ncbi.nlm.nih.gov | |
Description | Data deposited in or computed by PubChem | |
InChI Key |
DPMGLJUMNRDNMX-UHFFFAOYSA-N | |
Details | Computed by InChI 1.0.5 (PubChem release 2019.06.18) | |
Source | PubChem | |
URL | https://pubchem.ncbi.nlm.nih.gov | |
Description | Data deposited in or computed by PubChem | |
Canonical SMILES |
CC(C)(C)C1COC(=N1)C(C)(C)C2=NC(CO2)C(C)(C)C | |
Details | Computed by OEChem 2.1.5 (PubChem release 2019.06.18) | |
Source | PubChem | |
URL | https://pubchem.ncbi.nlm.nih.gov | |
Description | Data deposited in or computed by PubChem | |
Molecular Formula |
C17H30N2O2 | |
Details | Computed by PubChem 2.1 (PubChem release 2019.06.18) | |
Source | PubChem | |
URL | https://pubchem.ncbi.nlm.nih.gov | |
Description | Data deposited in or computed by PubChem | |
DSSTOX Substance ID |
DTXSID20927361 | |
Record name | 2,2'-(Propane-2,2-diyl)bis(4-tert-butyl-4,5-dihydro-1,3-oxazole) | |
Source | EPA DSSTox | |
URL | https://comptox.epa.gov/dashboard/DTXSID20927361 | |
Description | DSSTox provides a high quality public chemistry resource for supporting improved predictive toxicology. | |
Molecular Weight |
294.4 g/mol | |
Details | Computed by PubChem 2.1 (PubChem release 2021.05.07) | |
Source | PubChem | |
URL | https://pubchem.ncbi.nlm.nih.gov | |
Description | Data deposited in or computed by PubChem | |
CAS No. |
131833-93-7 | |
Record name | 2,2'-(Propane-2,2-diyl)bis(4-tert-butyl-4,5-dihydro-1,3-oxazole) | |
Source | EPA DSSTox | |
URL | https://comptox.epa.gov/dashboard/DTXSID20927361 | |
Description | DSSTox provides a high quality public chemistry resource for supporting improved predictive toxicology. | |
Retrosynthesis Analysis
AI-Powered Synthesis Planning: Our tool employs the Template_relevance Pistachio, Template_relevance Bkms_metabolic, Template_relevance Pistachio_ringbreaker, Template_relevance Reaxys, Template_relevance Reaxys_biocatalysis model, leveraging a vast database of chemical reactions to predict feasible synthetic routes.
One-Step Synthesis Focus: Specifically designed for one-step synthesis, it provides concise and direct routes for your target compounds, streamlining the synthesis process.
Accurate Predictions: Utilizing the extensive PISTACHIO, BKMS_METABOLIC, PISTACHIO_RINGBREAKER, REAXYS, REAXYS_BIOCATALYSIS database, our tool offers high-accuracy predictions, reflecting the latest in chemical research and data.
Strategy Settings
Precursor scoring | Relevance Heuristic |
---|---|
Min. plausibility | 0.01 |
Model | Template_relevance |
Template Set | Pistachio/Bkms_metabolic/Pistachio_ringbreaker/Reaxys/Reaxys_biocatalysis |
Top-N result to add to graph | 6 |
Feasible Synthetic Routes
Disclaimer and Information on In-Vitro Research Products
Please be aware that all articles and product information presented on BenchChem are intended solely for informational purposes. The products available for purchase on BenchChem are specifically designed for in-vitro studies, which are conducted outside of living organisms. In-vitro studies, derived from the Latin term "in glass," involve experiments performed in controlled laboratory settings using cells or tissues. It is important to note that these products are not categorized as medicines or drugs, and they have not received approval from the FDA for the prevention, treatment, or cure of any medical condition, ailment, or disease. We must emphasize that any form of bodily introduction of these products into humans or animals is strictly prohibited by law. It is essential to adhere to these guidelines to ensure compliance with legal and ethical standards in research and experimentation.