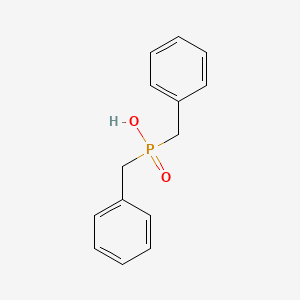
dibenzylphosphinic acid
Overview
Description
Dibenzylphosphinic acid is a chemical compound with the molecular formula C14H15O2P . It contains a total of 33 bonds, including 18 non-H bonds, 13 multiple bonds, 4 rotatable bonds, 1 double bond, 12 aromatic bonds, 2 six-membered rings, and 1 hydroxyl group .
Synthesis Analysis
This compound has been used in the synthesis of α-aminophosphonates using a multicomponent Kabachnik-Fields reaction . Another synthesis method involves the interaction of α-olefin vinylidene dimers with H3PO2 in an isopropanol medium at 90 °C .
Molecular Structure Analysis
The molecular structure of this compound consists of 15 Hydrogen atoms, 14 Carbon atoms, 2 Oxygen atoms, and 1 Phosphorous atom . The molecular weight is 246.242 Da .
Chemical Reactions Analysis
This compound has been used as an efficient and simple catalyst for the synthesis of α-aminophosphonates . It has also been used in the preparation of coordination polymers .
Scientific Research Applications
Disinfection By-products Formation
Dibenzylphosphinic acid's impact in water treatment is evident in studies on disinfection by-products. When chlorine dioxide is used as a disinfectant, this compound, as a part of organic matter in water, can influence the formation of these by-products. The research by Chang et al. (2001) indicates that different organic groups, including this compound, contribute to the varying levels of by-products during water disinfection processes (Chang et al., 2001).
Antioxidant and Anticholinergic Activities
Usnic acid, a this compound derivative, has been studied for its antioxidant and anticholinergic activities. Cetin Çakmak and Gülçin (2019) explored usnic acid's potential as a natural product with significant antioxidant properties, highlighting its efficacy in scavenging various radicals (Cetin Çakmak & Gülçin, 2019).
Chemical Synthesis and Flow Operations
This compound is utilized in microwave-assisted direct esterification and transesterification processes. Kiss, Henyecz, and Keglevich (2020) demonstrated its application in synthesizing optically active reagents, showing its importance in chemical synthesis and flow operations (Kiss et al., 2020).
Vanadium Complex Synthesis
In the field of coordination chemistry, this compound plays a crucial role in synthesizing vanadium complexes. Maass et al. (2016) discussed its use in creating trimeric and hexameric vanadium complexes, providing insights into the complex structures and potential applications in materials science (Maass et al., 2016).
Future Directions
The use of dibenzylphosphinic acid in the synthesis of α-aminophosphonates and coordination polymers suggests potential future directions in the field of organic synthesis . Additionally, the use of similar compounds in the extraction and separation of rare-earth elements suggests potential industrial applications .
Properties
IUPAC Name |
dibenzylphosphinic acid | |
---|---|---|
Details | Computed by Lexichem TK 2.7.0 (PubChem release 2021.05.07) | |
Source | PubChem | |
URL | https://pubchem.ncbi.nlm.nih.gov | |
Description | Data deposited in or computed by PubChem | |
InChI |
InChI=1S/C14H15O2P/c15-17(16,11-13-7-3-1-4-8-13)12-14-9-5-2-6-10-14/h1-10H,11-12H2,(H,15,16) | |
Details | Computed by InChI 1.0.6 (PubChem release 2021.05.07) | |
Source | PubChem | |
URL | https://pubchem.ncbi.nlm.nih.gov | |
Description | Data deposited in or computed by PubChem | |
InChI Key |
LGMGVCQVPSHUCO-UHFFFAOYSA-N | |
Details | Computed by InChI 1.0.6 (PubChem release 2021.05.07) | |
Source | PubChem | |
URL | https://pubchem.ncbi.nlm.nih.gov | |
Description | Data deposited in or computed by PubChem | |
Canonical SMILES |
C1=CC=C(C=C1)CP(=O)(CC2=CC=CC=C2)O | |
Details | Computed by OEChem 2.3.0 (PubChem release 2021.05.07) | |
Source | PubChem | |
URL | https://pubchem.ncbi.nlm.nih.gov | |
Description | Data deposited in or computed by PubChem | |
Molecular Formula |
C14H15O2P | |
Details | Computed by PubChem 2.1 (PubChem release 2021.05.07) | |
Source | PubChem | |
URL | https://pubchem.ncbi.nlm.nih.gov | |
Description | Data deposited in or computed by PubChem | |
Molecular Weight |
246.24 g/mol | |
Details | Computed by PubChem 2.1 (PubChem release 2021.05.07) | |
Source | PubChem | |
URL | https://pubchem.ncbi.nlm.nih.gov | |
Description | Data deposited in or computed by PubChem | |
Retrosynthesis Analysis
AI-Powered Synthesis Planning: Our tool employs the Template_relevance Pistachio, Template_relevance Bkms_metabolic, Template_relevance Pistachio_ringbreaker, Template_relevance Reaxys, Template_relevance Reaxys_biocatalysis model, leveraging a vast database of chemical reactions to predict feasible synthetic routes.
One-Step Synthesis Focus: Specifically designed for one-step synthesis, it provides concise and direct routes for your target compounds, streamlining the synthesis process.
Accurate Predictions: Utilizing the extensive PISTACHIO, BKMS_METABOLIC, PISTACHIO_RINGBREAKER, REAXYS, REAXYS_BIOCATALYSIS database, our tool offers high-accuracy predictions, reflecting the latest in chemical research and data.
Strategy Settings
Precursor scoring | Relevance Heuristic |
---|---|
Min. plausibility | 0.01 |
Model | Template_relevance |
Template Set | Pistachio/Bkms_metabolic/Pistachio_ringbreaker/Reaxys/Reaxys_biocatalysis |
Top-N result to add to graph | 6 |
Feasible Synthetic Routes
Q1: What is the structural characterization of dibenzylphosphinic acid, and how does this influence its applications?
A1: this compound features a phosphorus atom bonded to two benzyl groups (C6H5CH2-) and two oxygen atoms, one of which carries a hydrogen (forming the acidic -OH group). Its molecular formula is C14H15O2P, and it has a molecular weight of 246.25 g/mol. While specific spectroscopic data is not provided in the given abstracts, FTIR, Raman, and NMR spectroscopy are valuable tools for characterizing this compound []. The presence of both aromatic rings (from benzyl groups) and a phosphinic acid moiety contributes to its versatile reactivity. This allows this compound to act as a ligand in coordination chemistry [] and as a building block in materials science, particularly for enhancing flame retardancy in polymers [].
Q2: How does this compound interact with metals to form complexes, and what are the structural characteristics of these complexes?
A2: this compound acts as a bidentate ligand through its two oxygen atoms from the phosphinic acid group (O2P). This allows it to bridge multiple metal centers, leading to the formation of polynuclear complexes. For instance, it reacts with vanadium precursors to yield trimeric or hexameric vanadium clusters []. These clusters feature a central V3(μ3-O)O2 core where three vanadium ions are bridged by oxygen atoms. This compound coordinates to these vanadium ions through its O2P groups, creating a complex network of metal-oxygen-phosphorus bonds.
Q3: What is the significance of the aluminum-dibenzylphosphinic acid hybrid nanorods (AOPH-NR) and their impact on material properties?
A3: Aluminum-dibenzylphosphinic acid hybrid nanorods represent a unique class of materials with potential applications in flame retardancy []. These nanorods are synthesized by reacting aluminum hydroxide (ATH) with this compound (DBPA). The resulting AOPH-NR exhibit a highly hybrid structure and superior thermal stability compared to similar compounds synthesized with different phosphinic acids. When incorporated into epoxy resin, AOPH-NR significantly enhances its flame retardancy. This improvement is attributed to the unique morphology and thermal properties of AOPH-NR, which allow for efficient release and migration of phosphorus species during combustion, acting as a flame retardant mechanism. Additionally, the inclusion of AOPH-NR leads to an improvement in the mechanical properties of the epoxy resin [].
Q4: Can this compound be used as a starting material for synthesizing other organophosphorus compounds?
A4: While not directly discussed in the provided abstracts, this compound can potentially serve as a precursor for synthesizing other organophosphorus compounds. The reactivity of the phosphinic acid group, particularly the P-H bond, allows for various chemical transformations. These could include esterification, alkylation, or reactions with electrophiles, offering pathways to access diverse organophosphorus derivatives.
Q5: What analytical techniques are employed to study this compound and its derivatives?
A5: Various analytical techniques are essential for characterizing this compound and its derivatives. FTIR and Raman spectroscopy provide information about the vibrational modes of the molecule, revealing insights into its functional groups and bonding. NMR spectroscopy, particularly 1H and 31P NMR, helps determine the structure and purity of the compound. For metal complexes, single-crystal X-ray diffraction is a powerful tool to elucidate the three-dimensional arrangement of atoms and bonding patterns within the complex []. Thermal analysis techniques like TGA are crucial for understanding the thermal stability and decomposition behavior of these compounds, especially in the context of their flame-retardant properties [].
Disclaimer and Information on In-Vitro Research Products
Please be aware that all articles and product information presented on BenchChem are intended solely for informational purposes. The products available for purchase on BenchChem are specifically designed for in-vitro studies, which are conducted outside of living organisms. In-vitro studies, derived from the Latin term "in glass," involve experiments performed in controlled laboratory settings using cells or tissues. It is important to note that these products are not categorized as medicines or drugs, and they have not received approval from the FDA for the prevention, treatment, or cure of any medical condition, ailment, or disease. We must emphasize that any form of bodily introduction of these products into humans or animals is strictly prohibited by law. It is essential to adhere to these guidelines to ensure compliance with legal and ethical standards in research and experimentation.