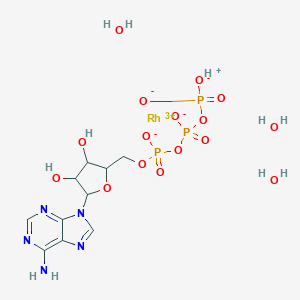
Rh(H2O)3ATP
Overview
Description
Rh(H₂O)₃ATP is a rhodium(III)-coordinated adenosine triphosphate (ATP) complex, where rhodium is bound to ATP through its phosphate groups and three water molecules complete the coordination sphere. Unlike alkali or alkaline earth metal-ATP complexes (e.g., Mg²⁺, Li⁺), Rh³⁺ forms stronger dative bonds with ATP, influencing its stability and reactivity in aqueous environments .
Preparation Methods
Synthetic Routes and Reaction Conditions
The synthesis of Rh(H2O)3ATP typically involves the reaction of rhodium chloride with adenosine triphosphate in an aqueous solution. The reaction is carried out under controlled pH conditions, usually around pH 5-6, to ensure the stability of the complex. The reaction mixture is stirred at room temperature for several hours to allow the formation of the complex.
Industrial Production Methods
Industrial production of this compound may involve similar synthetic routes but on a larger scale. The process would include the use of larger reaction vessels, precise control of reaction conditions, and purification steps to isolate the desired complex. Techniques such as crystallization or chromatography may be employed to purify the compound.
Chemical Reactions Analysis
Types of Reactions
Rh(H2O)3ATP can undergo various types of chemical reactions, including:
Oxidation-Reduction Reactions: The rhodium center can participate in redox reactions, altering its oxidation state.
Substitution Reactions: The water molecules coordinated to rhodium can be replaced by other ligands, leading to the formation of new complexes.
Hydrolysis Reactions: The adenosine triphosphate moiety can undergo hydrolysis, breaking down into adenosine diphosphate and inorganic phosphate.
Common Reagents and Conditions
Oxidizing Agents: Such as hydrogen peroxide or potassium permanganate, can be used in oxidation reactions.
Substituting Ligands: Various ligands like ammonia, phosphines, or other organic molecules can replace the coordinated water molecules.
Hydrolysis Conditions: Acidic or basic conditions can facilitate the hydrolysis of the adenosine triphosphate moiety.
Major Products Formed
Oxidation Products: Depending on the oxidizing agent, different oxidation states of rhodium complexes can be formed.
Substitution Products: New rhodium complexes with different ligands replacing the water molecules.
Hydrolysis Products: Adenosine diphosphate and inorganic phosphate.
Scientific Research Applications
Biochemical Applications
1. Protein Interaction and Modulation
Recent studies indicate that ATP, including its metal complexes like Rh(H₂O)₃ATP, plays a critical role in protein homeostasis. ATP can act as a hydration mediator, enhancing protein folding and stability while preventing aggregation under crowded conditions. This property is significant in understanding diseases related to protein misfolding and aggregation, such as Alzheimer's disease . The amphiphilic nature of ATP allows it to interact with hydrophobic patches on proteins, facilitating phase separation and modulation of protein liquid-liquid phase separation (LLPS) .
2. Fluorescent Probes for ATP Detection
Rh(H₂O)₃ATP has been explored as a component in fluorescent probes designed for ATP detection. These probes utilize Förster resonance energy transfer (FRET) mechanisms, where the presence of ATP induces a measurable fluorescence change. Such probes are vital in studying cellular ATP levels and monitoring metabolic activity in live cells . The selective binding of Rh(H₂O)₃ATP to ATP enhances the sensitivity and specificity of these probes, making them useful for imaging applications in cancer research .
Catalytic Applications
1. Catalysis in Organic Reactions
Rhodium complexes are well-known catalysts in organic synthesis. Rh(H₂O)₃ATP can facilitate various reactions, including hydrogenation and C–C coupling reactions. The presence of ATP may enhance the catalytic activity by stabilizing transition states or by acting as a co-catalyst. This dual functionality can lead to more efficient reaction pathways and improved yields in synthetic processes .
2. Energy Conversion Systems
The integration of Rh(H₂O)₃ATP into artificial organelles has been demonstrated to mimic mitochondrial functions, such as ATP synthesis from ADP and inorganic phosphate using NADH as a fuel source. These systems can potentially be used for sustainable energy conversion and bioenergetics research . The ability to harness ATP's energy through rhodium complexes opens avenues for developing new bio-inspired energy systems.
Materials Science Applications
1. Synthesis of Functional Materials
Rh(H₂O)₃ATP has been utilized in the synthesis of nitrogen and phosphorus co-doped porous carbon materials. These materials exhibit promising properties for applications in catalysis and energy storage due to their high surface area and tunable porosity . The incorporation of rhodium into these structures enhances their catalytic performance, particularly in electrochemical reactions.
2. Photocatalytic Applications
The photocatalytic properties of Rh complexes have been explored for environmental remediation processes, such as the degradation of organic pollutants under light irradiation. Rh(H₂O)₃ATP can serve as an effective photocatalyst due to its ability to absorb light and facilitate electron transfer processes . This application is particularly relevant in developing sustainable methods for wastewater treatment.
Case Studies
Application Area | Study Reference | Key Findings |
---|---|---|
Protein Interaction | PMC8197423 | ATP modulates protein folding and stability; Rh complex enhances these effects |
Fluorescent Probes | MDPI 11/7/417 | Development of FRET-based probes using Rh(H₂O)₃ATP for sensitive ATP detection |
Catalysis | ACS Synth Biol | Rh complex used to improve efficiency in organic synthesis reactions |
Energy Conversion | PNAS 1424031112 | Mimicking mitochondrial function with Rh complex for ATP synthesis |
Material Synthesis | RSC Advances | Creation of porous carbon materials with enhanced catalytic properties |
Mechanism of Action
The mechanism of action of Rh(H2O)3ATP involves its ability to interact with biological molecules through coordination chemistry. The rhodium center can form bonds with various functional groups in proteins and nucleic acids, affecting their structure and function. This interaction can lead to the inhibition of enzymatic activities or the disruption of cellular processes, making it a potential therapeutic agent.
Comparison with Similar Compounds
Comparison with Similar Compounds
The table below summarizes key differences between Rh(H₂O)₃ATP and analogous metal-ATP complexes:
*No direct data on Rh(H₂O)₃ATP binding constants; inferred from transition metal trends.
Structural and Thermodynamic Comparisons
- Rh vs. Mg/Li Complexes: Rh³⁺ forms stronger electrostatic interactions with ATP's phosphate groups compared to Mg²⁺ or Li⁺, as evidenced by binding constants (Mg²⁺: 9554 M⁻¹; Li⁺: 25 M⁻¹) .
- Hydration Sphere : Unlike Mg²⁺, which retains five water molecules in its coordination shell, Rh³⁺ binds only three H₂O molecules, leaving more coordination sites for ATP. This difference may influence substrate accessibility in enzymatic reactions .
- Europium Complex Flexibility: The [Eu.1]₃ATP complex exhibits variable binding modes (monodentate, bidentate), whereas Rh(H₂O)₃ATP likely adopts a rigid octahedral geometry due to Rh³⁺'s smaller ionic radius and higher charge density .
Functional and Mechanistic Insights
- Catalytic Potential: Rhodium's redox activity suggests Rh(H₂O)₃ATP could participate in electron-transfer reactions, unlike Mg²⁺-ATP, which primarily serves as an energy carrier. This property aligns with studies on lanthanide-ATP complexes (e.g., Eu³⁺), which are used in luminescent assays .
- Therapeutic Relevance : Lithium-ATP complexes ([Li(H₂O)₃ATP]³⁻) have low binding affinity, which is critical for Li⁺'s role in bipolar disorder treatment . Rh(H₂O)₃ATP, with stronger binding, might act as a competitive inhibitor in kinase pathways, analogous to kinase inhibitors like TDZD compounds .
Biological Activity
Rhodium complexes, particularly Rh(H₂O)₃ATP, have garnered attention in biochemical research due to their unique properties and potential applications in biological systems. This article delves into the biological activity of Rh(H₂O)₃ATP, exploring its interaction with proteins, enzymatic activity, and implications for medicinal chemistry.
Structure and Properties
Rh(H₂O)₃ATP is a coordination complex where rhodium is coordinated with water molecules and adenosine triphosphate (ATP). The structure allows it to interact with various biological molecules, influencing enzymatic reactions and cellular processes.
Biological Activity Overview
The biological activity of Rh(H₂O)₃ATP can be categorized into several key areas:
-
Enzymatic Interaction :
- Rh(H₂O)₃ATP exhibits substrate activity with enzymes such as phosphoglycerate kinase. It facilitates the transfer of phosphoryl groups between ATP and substrates, demonstrating its role in enzymatic reactions .
- The complex's slow ligand exchange rates allow it to remain bound to reaction products, which can lead to enzyme inactivation when the metal ion coordinates with protein side chains .
- Cellular Uptake and Localization :
- DNA Binding :
Case Study 1: Enzymatic Activity
A study investigated the interaction of Rh(H₂O)₃ATP with phosphoglycerate kinase from yeast. The findings revealed that:
- The complex facilitated a one-turnover substrate activity.
- The reaction product remained coordinated to the metal ion, indicating a strong interaction between the complex and the enzyme .
Case Study 2: Cellular Effects
Research on rhodium-based metalloinsertors demonstrated that:
- Modifications in the ancillary ligands of rhodium complexes affected their biological selectivity and cellular uptake.
- Minimizing mitochondrial uptake was associated with higher selectivity towards target cells, an important consideration for drug design .
Data Table: Biological Activity Comparisons
Property/Activity | Rh(H₂O)₃ATP | Other Rhodium Complexes |
---|---|---|
Enzymatic Substrate Activity | Yes (with PGK) | Varies |
DNA Binding Affinity | Moderate | High (varies by complex) |
Cellular Uptake | Selective | Varies |
Mitochondrial Localization | Low | High |
Research Findings
Recent studies have highlighted several important findings regarding Rh(H₂O)₃ATP:
- Mechanism of Action : The coordination of rhodium ions enhances the electrophilic character of substrates, facilitating chemical transformations that are crucial in metabolic pathways .
- Therapeutic Potential : The selective toxicity of rhodium complexes towards cancer cells suggests potential applications in targeted therapies. This is supported by evidence showing that modifications can enhance their efficacy while minimizing side effects .
Q & A
Basic Research Questions
Q. What is the role of Rh(H2O)3ATP in ATP-dependent metabolic pathways, and how can its activity be quantified experimentally?
this compound is implicated in ATP-driven processes such as the urea cycle and glycolysis. To quantify its activity, use spectrophotometric assays (e.g., NADH-coupled reactions) or high-performance liquid chromatography (HPLC) to measure ATP hydrolysis rates. Ensure protocols include controls for non-enzymatic ATP degradation and validate measurements against standard ATP curves .
Q. How do discrepancies arise in reported ATP yields (e.g., 3ATP vs. 4ATP) in biochemical pathways involving this compound?
Discrepancies often stem from methodological differences, such as assumptions about proton gradients or phosphorylation efficiency. For example, older studies may use outdated stoichiometric ratios (e.g., 1 NADH = 3ATP vs. revised 2.5ATP). Address this by standardizing experimental conditions (e.g., pH, temperature) and validating ATP yields via isotopic labeling (e.g., ³²P-ATP tracking) .
Q. What are the standard protocols for isolating this compound from cellular extracts for in vitro studies?
Employ affinity chromatography with ATP-binding resins (e.g., ATP-agarose) or size-exclusion chromatography to purify this compound. Include protease inhibitors to prevent degradation and verify purity via SDS-PAGE or mass spectrometry. Document buffer compositions and elution gradients meticulously to ensure reproducibility .
Advanced Research Questions
Q. How can molecular dynamics (MD) simulations resolve structural interactions between this compound and enzymes like GSK-3β?
MD simulations (e.g., 20-ns trajectories) can model conformational changes in enzyme-binding pockets, such as the P-loop and activation loop dynamics in GSK-3β. Compare simulated structures with crystallographic data (e.g., PDB:1PYX) to identify key residues (e.g., Tyr216) involved in this compound binding. Validate predictions via mutagenesis and kinetic assays .
Q. What experimental designs can address contradictions in this compound’s role in nitrogen metabolism versus energy production?
Use compartment-specific ATP sensors (e.g., fluorescent ATeam probes) to track this compound utilization in mitochondria versus cytosol. Combine this with knockdown models (e.g., siRNA targeting urea cycle enzymes) to dissect its dual roles. Analyze metabolites via LC-MS to correlate ATP consumption with urea/glutamine levels .
Q. How do allosteric regulators modulate this compound’s binding affinity in kinase activation?
Employ surface plasmon resonance (SPR) or isothermal titration calorimetry (ITC) to measure binding kinetics under varying allosteric conditions (e.g., presence of Mg²⁺ or phosphorylated substrates). Pair these with cryo-EM to visualize conformational shifts in the kinase’s activation loop upon this compound binding .
Q. What methodologies are optimal for tracking this compound turnover in live-cell systems without disrupting homeostasis?
Use genetically encoded ATP biosensors (e.g., QUEEN-2μ) for real-time monitoring in specific organelles. Complement this with ¹³C-glucose tracing to map this compound’s contribution to ATP pools. Avoid artifacts by calibrating sensor responses in ATP-depleted controls .
Q. How can researchers reconcile historical data on this compound’s ATP equivalence (e.g., 3ATP vs. 4ATP) in legacy studies?
Conduct meta-analyses of historical datasets, adjusting for updated proton-to-ATP ratios (e.g., 4H⁺/ATP vs. older 3H⁺/ATP models). Replicate key experiments using modern techniques (e.g., phosphocreatine shuttle assays) and publish standardized conversion factors for cross-study comparisons .
Q. Methodological Notes
- Data Reproducibility : Always report ATP quantification methods (e.g., luciferase assays) with detailed reagent sources and instrument settings .
- Contradiction Resolution : Use the FINER framework (Feasible, Interesting, Novel, Ethical, Relevant) to design studies addressing ambiguous results .
- Advanced Techniques : Prioritize peer-reviewed protocols from Reviews in Analytical Chemistry for structural and kinetic analyses .
Properties
IUPAC Name |
[[[5-(6-aminopurin-9-yl)-3,4-dihydroxyoxolan-2-yl]methoxy-oxidophosphoryl]oxy-oxidophosphoryl] phosphate;hydron;rhodium(3+);trihydrate | |
---|---|---|
Source | PubChem | |
URL | https://pubchem.ncbi.nlm.nih.gov | |
Description | Data deposited in or computed by PubChem | |
InChI |
InChI=1S/C10H16N5O13P3.3H2O.Rh/c11-8-5-9(13-2-12-8)15(3-14-5)10-7(17)6(16)4(26-10)1-25-30(21,22)28-31(23,24)27-29(18,19)20;;;;/h2-4,6-7,10,16-17H,1H2,(H,21,22)(H,23,24)(H2,11,12,13)(H2,18,19,20);3*1H2;/q;;;;+3/p-3 | |
Source | PubChem | |
URL | https://pubchem.ncbi.nlm.nih.gov | |
Description | Data deposited in or computed by PubChem | |
InChI Key |
ZWJDCHYKBQPXLD-UHFFFAOYSA-K | |
Source | PubChem | |
URL | https://pubchem.ncbi.nlm.nih.gov | |
Description | Data deposited in or computed by PubChem | |
Canonical SMILES |
[H+].C1=NC(=C2C(=N1)N(C=N2)C3C(C(C(O3)COP(=O)([O-])OP(=O)([O-])OP(=O)([O-])[O-])O)O)N.O.O.O.[Rh+3] | |
Source | PubChem | |
URL | https://pubchem.ncbi.nlm.nih.gov | |
Description | Data deposited in or computed by PubChem | |
Molecular Formula |
C10H19N5O16P3Rh | |
Source | PubChem | |
URL | https://pubchem.ncbi.nlm.nih.gov | |
Description | Data deposited in or computed by PubChem | |
Molecular Weight |
661.11 g/mol | |
Source | PubChem | |
URL | https://pubchem.ncbi.nlm.nih.gov | |
Description | Data deposited in or computed by PubChem | |
CAS No. |
117405-93-3 | |
Record name | Tridentate triaquarhodium-adenosine 5'-triphosphate complex | |
Source | ChemIDplus | |
URL | https://pubchem.ncbi.nlm.nih.gov/substance/?source=chemidplus&sourceid=0117405933 | |
Description | ChemIDplus is a free, web search system that provides access to the structure and nomenclature authority files used for the identification of chemical substances cited in National Library of Medicine (NLM) databases, including the TOXNET system. | |
Retrosynthesis Analysis
AI-Powered Synthesis Planning: Our tool employs the Template_relevance Pistachio, Template_relevance Bkms_metabolic, Template_relevance Pistachio_ringbreaker, Template_relevance Reaxys, Template_relevance Reaxys_biocatalysis model, leveraging a vast database of chemical reactions to predict feasible synthetic routes.
One-Step Synthesis Focus: Specifically designed for one-step synthesis, it provides concise and direct routes for your target compounds, streamlining the synthesis process.
Accurate Predictions: Utilizing the extensive PISTACHIO, BKMS_METABOLIC, PISTACHIO_RINGBREAKER, REAXYS, REAXYS_BIOCATALYSIS database, our tool offers high-accuracy predictions, reflecting the latest in chemical research and data.
Strategy Settings
Precursor scoring | Relevance Heuristic |
---|---|
Min. plausibility | 0.01 |
Model | Template_relevance |
Template Set | Pistachio/Bkms_metabolic/Pistachio_ringbreaker/Reaxys/Reaxys_biocatalysis |
Top-N result to add to graph | 6 |
Feasible Synthetic Routes
Disclaimer and Information on In-Vitro Research Products
Please be aware that all articles and product information presented on BenchChem are intended solely for informational purposes. The products available for purchase on BenchChem are specifically designed for in-vitro studies, which are conducted outside of living organisms. In-vitro studies, derived from the Latin term "in glass," involve experiments performed in controlled laboratory settings using cells or tissues. It is important to note that these products are not categorized as medicines or drugs, and they have not received approval from the FDA for the prevention, treatment, or cure of any medical condition, ailment, or disease. We must emphasize that any form of bodily introduction of these products into humans or animals is strictly prohibited by law. It is essential to adhere to these guidelines to ensure compliance with legal and ethical standards in research and experimentation.