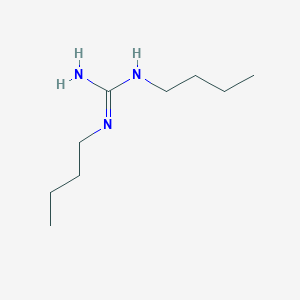
Guanidine, N,N'-dibutyl-
Overview
Description
Guanidine, N,N’-dibutyl- is a type of guanidine derivative where the guanidine core is substituted with two butyl groups. Guanidines are known for their high basicity and ability to form strong hydrogen bonds. These properties make them valuable in various biological and chemical applications, including pharmaceuticals, organocatalysis, and as precursors for the synthesis of heterocycles .
Mechanism of Action
Target of Action
Guanidine, a strong organic base, primarily targets the Aldehyde dehydrogenase, mitochondrial in humans . This enzyme plays a crucial role in the metabolism of aldehydes. Guanidine also interacts with other targets such as Ribonuclease pancreatic, DNA, Disks large homolog 4, Lysozyme, Guanidinoacetate N-methyltransferase, and Arginase .
Mode of Action
Guanidine acts by enhancing the release of acetylcholine following a nerve impulse . It also appears to slow the rates of depolarization and repolarization of muscle cell membranes . This interaction with its targets leads to changes in the physiological functions of these targets, affecting the overall metabolic processes in the body.
Biochemical Pathways
The biochemical pathways affected by Guanidine are primarily related to protein metabolism. Guanidine is found in the urine as a normal product of protein metabolism . It is also used in laboratory research as a protein denaturant . The exact biochemical pathways and their downstream effects are complex and depend on the specific targets and the physiological context.
Pharmacokinetics
Guanidine is rapidly absorbed and distributed in the body . The half-life of Guanidine is approximately 7-8 hours . These properties impact the bioavailability of Guanidine, influencing its therapeutic effects.
Result of Action
The primary result of Guanidine’s action is the reduction of the symptoms of muscle weakness and easy fatigability associated with the myasthenic syndrome of Eaton-Lambert . . The molecular and cellular effects of Guanidine’s action are complex and depend on the specific targets and the physiological context.
Action Environment
The action, efficacy, and stability of Guanidine can be influenced by various environmental factors. These may include the physiological pH, which affects the existence of Guanidine primarily as guanidium ions . Other factors such as temperature, presence of other substances, and specific conditions within the body can also influence the action of Guanidine.
Biochemical Analysis
Biochemical Properties
Guanidine, N,N’-dibutyl- is involved in various biochemical reactions. It interacts with several enzymes, proteins, and other biomolecules. The guanidine molecule is planar due to the conjugation between the lone pairs of the nitrogen atoms and the imine double bond . This planarity often determines the conformation of substituted guanidinium species as well as the interaction with aromatic systems in biological environments such as amino acids and nucleic acid bases .
Cellular Effects
The effects of Guanidine, N,N’-dibutyl- on various types of cells and cellular processes are diverse. For instance, guanidines, except for arginine and guanidine, induce seizures and convulsions in rat, rabbit, and cat by intracisternal injection .
Molecular Mechanism
The molecular mechanism of action of Guanidine, N,N’-dibutyl- involves both nucleophilic and electrophilic characters . The aminal function of guanidine acts as an N-nucleophile in the additions of unsaturated compounds .
Temporal Effects in Laboratory Settings
It is known that guanidine and its derivatives play a crucial role in the metabolism of living organisms .
Dosage Effects in Animal Models
The effects of Guanidine, N,N’-dibutyl- vary with different dosages in animal models. For instance, guanidine is used to treat muscle weakness and fatigue associated with the myasthenic complications of Eaton-Lambert syndrome .
Metabolic Pathways
Guanidine, N,N’-dibutyl- is involved in several metabolic pathways. Guanidine and its derivatives are prevalent in natural environments and are thought to be produced by a variety of microorganisms .
Transport and Distribution
The transport and distribution of Guanidine, N,N’-dibutyl- within cells and tissues are complex processes. Guanidines are exported from the bacterial cell by Gdx transporters .
Subcellular Localization
It is known that guanidines can form hydrogen bonds, and their planarity and high basicity make this functional group a very versatile one for compounds with biological activity .
Preparation Methods
Synthetic Routes and Reaction Conditions: The synthesis of N,N’-dibutylguanidine can be achieved through several methods. One common approach involves the reaction of butylamine with cyanamide under acidic conditions to form the corresponding guanidine derivative. Another method includes the use of thiourea derivatives as guanidylating agents, where butylamine reacts with N,N’-bis-tert-butoxycarbonylthiourea in the presence of a base like triethylamine and a catalyst such as mercury chloride .
Industrial Production Methods: Industrial production of N,N’-dibutylguanidine typically involves large-scale reactions using similar methods as described above. The choice of method depends on factors such as yield, cost, and environmental considerations. The use of transition metal catalysts and polymer-supported guanidylation are also explored to enhance efficiency and scalability .
Chemical Reactions Analysis
Types of Reactions: N,N’-dibutylguanidine undergoes various chemical reactions, including:
Oxidation: It can be oxidized to form corresponding guanidinium salts.
Reduction: Reduction reactions can convert it back to the corresponding amine and cyanamide.
Substitution: It can participate in nucleophilic substitution reactions, where the butyl groups can be replaced by other substituents.
Common Reagents and Conditions:
Oxidation: Common oxidizing agents include hydrogen peroxide and peracids.
Reduction: Reducing agents like lithium aluminum hydride or sodium borohydride are used.
Substitution: Nucleophiles such as halides or alkoxides can be used under basic conditions.
Major Products:
Oxidation: Guanidinium salts.
Reduction: Butylamine and cyanamide.
Substitution: Various substituted guanidines depending on the nucleophile used.
Scientific Research Applications
N,N’-dibutylguanidine has several scientific research applications:
Chemistry: Used as a catalyst in organic synthesis and as a building block for the synthesis of more complex molecules.
Biology: Investigated for its potential as a DNA minor groove binder and kinase inhibitor.
Medicine: Explored for its therapeutic potential in treating diseases related to enzyme inhibition.
Industry: Utilized in the production of polymers and as an additive in lubricants and coatings.
Comparison with Similar Compounds
- N,N’-dimethylguanidine
- N,N’-diethylguanidine
- N,N’-dipropylguanidine
Comparison: N,N’-dibutylguanidine is unique due to its longer butyl chains, which can influence its solubility, reactivity, and interaction with biological targets. Compared to its shorter-chain analogs, it may exhibit different pharmacokinetic properties and binding affinities, making it suitable for specific applications where these properties are advantageous .
Biological Activity
Guanidine, N,N'-dibutyl- (DBuG) is a derivative of guanidine that has garnered attention for its diverse biological activities. This article explores its mechanisms of action, therapeutic potential, and relevant research findings.
Chemical Structure and Properties
DBuG is characterized by the molecular formula , featuring two butyl groups attached to the nitrogen atoms in the guanidine structure. Its strong basicity and steric hindrance from the bulky butyl groups make it a unique compound for various applications in chemistry and biology.
DBuG exhibits several mechanisms of action that contribute to its biological activity:
- DNA Binding : DBuG acts as a minor groove binder, which may influence gene expression and cellular processes by stabilizing specific DNA conformations.
- Kinase Inhibition : It has been identified as a kinase inhibitor, potentially affecting signaling pathways involved in cell proliferation and survival.
- Neurotransmitter Modulation : The compound enhances the release of acetylcholine following a nerve impulse, influencing neuromuscular transmission. Additionally, it can modulate the activity of sigma receptors implicated in various neurological conditions .
Biological Activities
Research indicates that DBuG exhibits several notable biological activities:
Case Studies and Research Findings
Several studies have investigated the biological activity of DBuG:
- Sigma Receptor Binding Studies : Research demonstrated that DBuG competes effectively with known sigma ligands for binding sites in brain tissues, suggesting its potential role in modulating psychiatric conditions .
- In Vitro Studies on Antimicrobial Activity : Preliminary studies indicated that DBuG may disrupt bacterial membranes, contributing to its antimicrobial properties. However, comprehensive data on its effectiveness against specific strains are still needed .
- Neuropharmacological Assessments : In animal models, DBuG exhibited significant effects on behavior indicative of its potential as a treatment for anxiety and depression. These findings warrant further exploration into its therapeutic applications .
Data Summary
The following table summarizes key findings related to the biological activity of DBuG:
Properties
IUPAC Name |
1,2-dibutylguanidine | |
---|---|---|
Source | PubChem | |
URL | https://pubchem.ncbi.nlm.nih.gov | |
Description | Data deposited in or computed by PubChem | |
InChI |
InChI=1S/C9H21N3/c1-3-5-7-11-9(10)12-8-6-4-2/h3-8H2,1-2H3,(H3,10,11,12) | |
Source | PubChem | |
URL | https://pubchem.ncbi.nlm.nih.gov | |
Description | Data deposited in or computed by PubChem | |
InChI Key |
HXEJAEDEMWCUTP-UHFFFAOYSA-N | |
Source | PubChem | |
URL | https://pubchem.ncbi.nlm.nih.gov | |
Description | Data deposited in or computed by PubChem | |
Canonical SMILES |
CCCCNC(=NCCCC)N | |
Source | PubChem | |
URL | https://pubchem.ncbi.nlm.nih.gov | |
Description | Data deposited in or computed by PubChem | |
Molecular Formula |
C9H21N3 | |
Source | PubChem | |
URL | https://pubchem.ncbi.nlm.nih.gov | |
Description | Data deposited in or computed by PubChem | |
DSSTOX Substance ID |
DTXSID90563856 | |
Record name | N,N''-Dibutylguanidine | |
Source | EPA DSSTox | |
URL | https://comptox.epa.gov/dashboard/DTXSID90563856 | |
Description | DSSTox provides a high quality public chemistry resource for supporting improved predictive toxicology. | |
Molecular Weight |
171.28 g/mol | |
Source | PubChem | |
URL | https://pubchem.ncbi.nlm.nih.gov | |
Description | Data deposited in or computed by PubChem | |
CAS No. |
34331-58-3, 57028-96-3 | |
Record name | N,N''-Dibutylguanidine | |
Source | EPA DSSTox | |
URL | https://comptox.epa.gov/dashboard/DTXSID90563856 | |
Description | DSSTox provides a high quality public chemistry resource for supporting improved predictive toxicology. | |
Record name | poly(hexamethyleneguanide) hydrochloride | |
Source | European Chemicals Agency (ECHA) | |
URL | https://echa.europa.eu/information-on-chemicals | |
Description | The European Chemicals Agency (ECHA) is an agency of the European Union which is the driving force among regulatory authorities in implementing the EU's groundbreaking chemicals legislation for the benefit of human health and the environment as well as for innovation and competitiveness. | |
Explanation | Use of the information, documents and data from the ECHA website is subject to the terms and conditions of this Legal Notice, and subject to other binding limitations provided for under applicable law, the information, documents and data made available on the ECHA website may be reproduced, distributed and/or used, totally or in part, for non-commercial purposes provided that ECHA is acknowledged as the source: "Source: European Chemicals Agency, http://echa.europa.eu/". Such acknowledgement must be included in each copy of the material. ECHA permits and encourages organisations and individuals to create links to the ECHA website under the following cumulative conditions: Links can only be made to webpages that provide a link to the Legal Notice page. | |
Synthesis routes and methods
Procedure details
Disclaimer and Information on In-Vitro Research Products
Please be aware that all articles and product information presented on BenchChem are intended solely for informational purposes. The products available for purchase on BenchChem are specifically designed for in-vitro studies, which are conducted outside of living organisms. In-vitro studies, derived from the Latin term "in glass," involve experiments performed in controlled laboratory settings using cells or tissues. It is important to note that these products are not categorized as medicines or drugs, and they have not received approval from the FDA for the prevention, treatment, or cure of any medical condition, ailment, or disease. We must emphasize that any form of bodily introduction of these products into humans or animals is strictly prohibited by law. It is essential to adhere to these guidelines to ensure compliance with legal and ethical standards in research and experimentation.