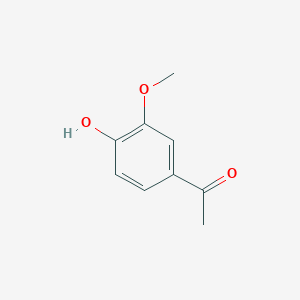
Acetovanillone
Overview
Description
It was first described by Oswald Schmiedeberg, a German pharmacologist, in 1883 and later isolated from the root of Canadian hemp (Apocynum cannabinum) by Horace Finnemore in 1908 . Apocynin has been extensively studied for its pharmacological properties, particularly its anti-inflammatory capabilities .
Mechanism of Action
Target of Action
Acetovanillone, also known as apocynin, primarily targets the NADPH oxidase enzyme . This enzyme plays a crucial role in the immune system by reducing O2 to superoxide (O2–•), which is used to kill bacteria and fungi .
Mode of Action
Apocynin acts as an inhibitor of NADPH oxidase activity, effectively preventing the production of superoxide in human agranulocytes or neutrophilic granulocytes . Due to the selectivity of its inhibition, apocynin can be widely used as an inhibitor of NADPH oxidase without interfering in other aspects of the immune system .
Biochemical Pathways
The biochemical pathway of this compound involves the hydroxyphenylethanone (Hpe) pathway . This pathway proceeds via phosphorylation and carboxylation, before β-elimination yields vanillate from this compound . The kinase, HpeHI, phosphorylates this compound . Furthermore, HpeCBA, a biotin-dependent enzyme, catalyzes the ATP-dependent carboxylation of 4-phospho-acetovanillone .
Pharmacokinetics
The ADME properties of this compound are yet to be fully explored. It has been shown that this compound has good admet and drug likeness attributes . These properties can impact the bioavailability of the compound, influencing its effectiveness in the body.
Result of Action
The action of this compound results in the reduction of reactive oxygen species concentrations, which has been observed in studies involving asthmatics . This suggests that the compound has anti-inflammatory capabilities due to its ability to selectively prevent the formation of free radicals, oxygen ions, and peroxides in the body .
Action Environment
The action, efficacy, and stability of this compound can be influenced by various environmental factors. For instance, the activity of the compound can be affected by the presence of different metal ions at varying concentrations . Additionally, the compound’s action can be influenced by the pH and temperature of its environment .
Biochemical Analysis
Biochemical Properties
Acetovanillone plays a crucial role in various biochemical reactions. It interacts with several enzymes and proteins, facilitating its conversion into other valuable compounds. For instance, in the bacterium Sphingobium sp. strain SYK-6, this compound is phosphorylated by the enzyme AcvAB and subsequently dephosphorylated by AcvF. The resulting product is then carboxylated by AcvCDE, leading to the formation of vanilloyl acetic acid . These interactions highlight the importance of this compound in microbial catabolic pathways, enabling the transformation of lignin-derived compounds into value-added chemicals.
Cellular Effects
This compound influences various cellular processes, including cell signaling pathways, gene expression, and cellular metabolism. In bacterial cells, this compound is metabolized through specific pathways that involve phosphorylation and carboxylation. These processes are essential for the growth and survival of bacteria that utilize this compound as a carbon source . Additionally, this compound’s impact on gene expression and cellular metabolism underscores its significance in microbial biocatalysis and lignin valorization.
Molecular Mechanism
The molecular mechanism of this compound involves several key steps. Initially, this compound is phosphorylated by specific kinases, such as HpeHI, which adds a phosphate group to the molecule. This phosphorylated intermediate is then carboxylated by biotin-dependent enzymes like HpeCBA, converting it into vanillate. The final step involves the dephosphorylation of the carboxylated product by enzymes such as HpeD . These molecular interactions demonstrate how this compound is transformed into valuable intermediates through a series of enzymatic reactions.
Temporal Effects in Laboratory Settings
In laboratory settings, the effects of this compound can change over time due to its stability and degradation. Studies have shown that this compound remains relatively stable under controlled conditions, allowing for its sustained use in biochemical reactions. Prolonged exposure to environmental factors such as light and temperature can lead to its degradation, affecting its efficacy in long-term experiments . Understanding these temporal effects is crucial for optimizing the use of this compound in various applications.
Dosage Effects in Animal Models
The effects of this compound vary with different dosages in animal models. At low doses, this compound has been shown to have minimal toxic effects, making it suitable for use in biochemical studies. At higher doses, this compound can exhibit toxic or adverse effects, including disruptions in cellular metabolism and gene expression . These dosage-dependent effects highlight the importance of determining the optimal concentration of this compound for specific applications.
Metabolic Pathways
This compound is involved in several metabolic pathways, particularly in the degradation of lignin-derived compounds. In bacteria, this compound is converted into vanillate through a series of enzymatic reactions, including phosphorylation, carboxylation, and dephosphorylation . These metabolic pathways are essential for the efficient utilization of this compound as a carbon source, enabling the production of valuable intermediates for industrial applications.
Transport and Distribution
Within cells, this compound is transported and distributed through specific transporters and binding proteins. These transporters facilitate the movement of this compound across cellular membranes, ensuring its availability for biochemical reactions. Additionally, binding proteins may interact with this compound, influencing its localization and accumulation within cells . Understanding these transport and distribution mechanisms is crucial for optimizing the use of this compound in various applications.
Subcellular Localization
This compound’s subcellular localization plays a significant role in its activity and function. Targeting signals and post-translational modifications can direct this compound to specific compartments or organelles within the cell. For instance, this compound may be localized to the cytoplasm or specific organelles involved in its metabolism
Preparation Methods
Synthetic Routes and Reaction Conditions: Apocynin can be synthesized through various methods. One common synthetic route involves the methylation of 4-hydroxyacetophenone using dimethyl sulfate in the presence of a base such as potassium carbonate. The reaction is typically carried out in an organic solvent like acetone .
Industrial Production Methods: Industrial production of apocynin often involves the extraction from plant sources such as Picrorhiza kurroa, a small plant that grows at high altitudes in the western Himalayas . The extraction process includes drying and grinding the plant material, followed by solvent extraction using alcohol or other suitable solvents.
Chemical Reactions Analysis
Types of Reactions: Apocynin undergoes various chemical reactions, including oxidation, reduction, and substitution reactions.
Common Reagents and Conditions:
Oxidation: Apocynin can be oxidized using reagents like hydrogen peroxide in the presence of peroxidase.
Reduction: Reduction of apocynin can be achieved using reducing agents such as sodium borohydride.
Substitution: Substitution reactions involving apocynin often use halogenating agents like bromine or chlorine.
Major Products: The major products formed from these reactions include various derivatives of apocynin, such as its nitrone derivative, which has shown improved pharmacokinetic behavior .
Scientific Research Applications
Apocynin has a wide range of scientific research applications:
Comparison with Similar Compounds
Properties
IUPAC Name |
1-(4-hydroxy-3-methoxyphenyl)ethanone | |
---|---|---|
Source | PubChem | |
URL | https://pubchem.ncbi.nlm.nih.gov | |
Description | Data deposited in or computed by PubChem | |
InChI |
InChI=1S/C9H10O3/c1-6(10)7-3-4-8(11)9(5-7)12-2/h3-5,11H,1-2H3 | |
Source | PubChem | |
URL | https://pubchem.ncbi.nlm.nih.gov | |
Description | Data deposited in or computed by PubChem | |
InChI Key |
DFYRUELUNQRZTB-UHFFFAOYSA-N | |
Source | PubChem | |
URL | https://pubchem.ncbi.nlm.nih.gov | |
Description | Data deposited in or computed by PubChem | |
Canonical SMILES |
CC(=O)C1=CC(=C(C=C1)O)OC | |
Source | PubChem | |
URL | https://pubchem.ncbi.nlm.nih.gov | |
Description | Data deposited in or computed by PubChem | |
Molecular Formula |
C9H10O3 | |
Source | PubChem | |
URL | https://pubchem.ncbi.nlm.nih.gov | |
Description | Data deposited in or computed by PubChem | |
DSSTOX Substance ID |
DTXSID7060097 | |
Record name | Acetovanillone | |
Source | EPA DSSTox | |
URL | https://comptox.epa.gov/dashboard/DTXSID7060097 | |
Description | DSSTox provides a high quality public chemistry resource for supporting improved predictive toxicology. | |
Molecular Weight |
166.17 g/mol | |
Source | PubChem | |
URL | https://pubchem.ncbi.nlm.nih.gov | |
Description | Data deposited in or computed by PubChem | |
CAS No. |
498-02-2 | |
Record name | Acetovanillone | |
Source | CAS Common Chemistry | |
URL | https://commonchemistry.cas.org/detail?cas_rn=498-02-2 | |
Description | CAS Common Chemistry is an open community resource for accessing chemical information. Nearly 500,000 chemical substances from CAS REGISTRY cover areas of community interest, including common and frequently regulated chemicals, and those relevant to high school and undergraduate chemistry classes. This chemical information, curated by our expert scientists, is provided in alignment with our mission as a division of the American Chemical Society. | |
Explanation | The data from CAS Common Chemistry is provided under a CC-BY-NC 4.0 license, unless otherwise stated. | |
Record name | Acetovanillone | |
Source | ChemIDplus | |
URL | https://pubchem.ncbi.nlm.nih.gov/substance/?source=chemidplus&sourceid=0000498022 | |
Description | ChemIDplus is a free, web search system that provides access to the structure and nomenclature authority files used for the identification of chemical substances cited in National Library of Medicine (NLM) databases, including the TOXNET system. | |
Record name | Apocynin | |
Source | DrugBank | |
URL | https://www.drugbank.ca/drugs/DB12618 | |
Description | The DrugBank database is a unique bioinformatics and cheminformatics resource that combines detailed drug (i.e. chemical, pharmacological and pharmaceutical) data with comprehensive drug target (i.e. sequence, structure, and pathway) information. | |
Explanation | Creative Common's Attribution-NonCommercial 4.0 International License (http://creativecommons.org/licenses/by-nc/4.0/legalcode) | |
Record name | Acetovanillone | |
Source | DTP/NCI | |
URL | https://dtp.cancer.gov/dtpstandard/servlet/dwindex?searchtype=NSC&outputformat=html&searchlist=209524 | |
Description | The NCI Development Therapeutics Program (DTP) provides services and resources to the academic and private-sector research communities worldwide to facilitate the discovery and development of new cancer therapeutic agents. | |
Explanation | Unless otherwise indicated, all text within NCI products is free of copyright and may be reused without our permission. Credit the National Cancer Institute as the source. | |
Record name | Acetovanillone | |
Source | DTP/NCI | |
URL | https://dtp.cancer.gov/dtpstandard/servlet/dwindex?searchtype=NSC&outputformat=html&searchlist=2146 | |
Description | The NCI Development Therapeutics Program (DTP) provides services and resources to the academic and private-sector research communities worldwide to facilitate the discovery and development of new cancer therapeutic agents. | |
Explanation | Unless otherwise indicated, all text within NCI products is free of copyright and may be reused without our permission. Credit the National Cancer Institute as the source. | |
Record name | Ethanone, 1-(4-hydroxy-3-methoxyphenyl)- | |
Source | EPA Chemicals under the TSCA | |
URL | https://www.epa.gov/chemicals-under-tsca | |
Description | EPA Chemicals under the Toxic Substances Control Act (TSCA) collection contains information on chemicals and their regulations under TSCA, including non-confidential content from the TSCA Chemical Substance Inventory and Chemical Data Reporting. | |
Record name | Acetovanillone | |
Source | EPA DSSTox | |
URL | https://comptox.epa.gov/dashboard/DTXSID7060097 | |
Description | DSSTox provides a high quality public chemistry resource for supporting improved predictive toxicology. | |
Record name | 4'-hydroxy-3'-methoxyacetophenone | |
Source | European Chemicals Agency (ECHA) | |
URL | https://echa.europa.eu/substance-information/-/substanceinfo/100.007.141 | |
Description | The European Chemicals Agency (ECHA) is an agency of the European Union which is the driving force among regulatory authorities in implementing the EU's groundbreaking chemicals legislation for the benefit of human health and the environment as well as for innovation and competitiveness. | |
Explanation | Use of the information, documents and data from the ECHA website is subject to the terms and conditions of this Legal Notice, and subject to other binding limitations provided for under applicable law, the information, documents and data made available on the ECHA website may be reproduced, distributed and/or used, totally or in part, for non-commercial purposes provided that ECHA is acknowledged as the source: "Source: European Chemicals Agency, http://echa.europa.eu/". Such acknowledgement must be included in each copy of the material. ECHA permits and encourages organisations and individuals to create links to the ECHA website under the following cumulative conditions: Links can only be made to webpages that provide a link to the Legal Notice page. | |
Record name | APOCYNIN | |
Source | FDA Global Substance Registration System (GSRS) | |
URL | https://gsrs.ncats.nih.gov/ginas/app/beta/substances/B6J7B9UDTR | |
Description | The FDA Global Substance Registration System (GSRS) enables the efficient and accurate exchange of information on what substances are in regulated products. Instead of relying on names, which vary across regulatory domains, countries, and regions, the GSRS knowledge base makes it possible for substances to be defined by standardized, scientific descriptions. | |
Explanation | Unless otherwise noted, the contents of the FDA website (www.fda.gov), both text and graphics, are not copyrighted. They are in the public domain and may be republished, reprinted and otherwise used freely by anyone without the need to obtain permission from FDA. Credit to the U.S. Food and Drug Administration as the source is appreciated but not required. | |
Retrosynthesis Analysis
AI-Powered Synthesis Planning: Our tool employs the Template_relevance Pistachio, Template_relevance Bkms_metabolic, Template_relevance Pistachio_ringbreaker, Template_relevance Reaxys, Template_relevance Reaxys_biocatalysis model, leveraging a vast database of chemical reactions to predict feasible synthetic routes.
One-Step Synthesis Focus: Specifically designed for one-step synthesis, it provides concise and direct routes for your target compounds, streamlining the synthesis process.
Accurate Predictions: Utilizing the extensive PISTACHIO, BKMS_METABOLIC, PISTACHIO_RINGBREAKER, REAXYS, REAXYS_BIOCATALYSIS database, our tool offers high-accuracy predictions, reflecting the latest in chemical research and data.
Strategy Settings
Precursor scoring | Relevance Heuristic |
---|---|
Min. plausibility | 0.01 |
Model | Template_relevance |
Template Set | Pistachio/Bkms_metabolic/Pistachio_ringbreaker/Reaxys/Reaxys_biocatalysis |
Top-N result to add to graph | 6 |
Feasible Synthetic Routes
Q1: How does acetovanillone exert its effects within cells?
A1: this compound is recognized as an inhibitor of NADPH (Nicotinamide adenine dinucleotide phosphate) oxidase activity, an enzyme complex responsible for generating reactive oxygen species (ROS) [, , ]. By inhibiting NADPH oxidase, this compound effectively suppresses the production of superoxide, a type of ROS, and mitigates oxidative stress [, , ].
Q2: What are the downstream consequences of this compound's interaction with NADPH oxidase?
A2: The inhibition of NADPH oxidase by this compound leads to a decrease in ROS production. This reduction in oxidative stress has been linked to several beneficial effects, including:
- Reduced Inflammation: this compound has shown promising anti-inflammatory effects in various models of brain injury [, ] and acute lung injury [].
- Protection against Aneurysm Progression: Studies suggest that this compound attenuates aneurysm formation by decreasing matrix metalloproteinase activation in a mouse model of Marfan syndrome [].
- Potential Anti-cancer Effects: this compound derivatives have demonstrated the ability to inhibit the migration of breast cancer cells [].
- Protection against Cyclophosphamide-Induced Cardiotoxicity: Studies have shown that this compound can prevent heart damage caused by the chemotherapy drug cyclophosphamide [].
Q3: What is the molecular formula and weight of this compound?
A3: this compound has the molecular formula C9H10O3 and a molecular weight of 166.17 g/mol.
Q4: What are the key spectroscopic features of this compound?
A4: Spectroscopic analyses like FTIR, 1H NMR, and mass spectrometry are crucial for identifying and characterizing this compound. Its FTIR spectrum resembles that of apocynin, particularly in the fingerprint region, while 1H NMR analysis confirms the loss of one hydrogen atom from each apocynin molecule during its formation []. Mass spectrometry, particularly negative ion atmospheric pressure chemical ionization (APCI) LCMS, further confirms the presence of this compound [].
Q5: Can this compound act as a catalyst in chemical reactions?
A5: While this compound itself is not typically used as a catalyst, it can be a product of catalytic reactions involving lignin. For instance, the catalytic wet air oxidation (CWAO) of lignin can yield this compound []. Additionally, this compound can be further oxidized to vanillin using catalysts like LaFeO3 [].
Q6: What are the potential applications of this compound derived from lignin?
A6: this compound, being a lignin-derived compound, holds potential as a platform chemical for various applications, including the production of vanillin, a flavoring agent, through catalytic oxidation [].
Q7: How is computational chemistry used to study this compound?
A7: Molecular docking simulations have been employed to investigate the interactions between this compound and specific targets, such as Keap1, a protein involved in regulating oxidative stress response []. These simulations provide insights into the binding affinities and potential mechanisms of action.
Q8: How do structural modifications of this compound influence its activity?
A8: Studies exploring this compound derivatives, particularly benzyl ethers, have shown that these modifications can impact biological activity []. While some derivatives displayed antileishmanial activity, a greater number exhibited antibacterial properties [].
Q9: How is this compound typically quantified and analyzed?
A9: Various analytical techniques are employed to determine and quantify this compound in different matrices. These include:
- High-Performance Liquid Chromatography (HPLC): HPLC is commonly used to separate and quantify this compound, particularly in complex mixtures like aged wine brandies [].
- Gas Chromatography-Mass Spectrometry (GC-MS): This technique is valuable for identifying and quantifying this compound and its metabolites in biological samples [].
- Gas Chromatography-Flame Ionization Detector (GC-FID): This method is utilized to analyze the chemical composition of essential oils, some of which contain this compound [].
Q10: What is known about the environmental fate of this compound?
A10: this compound can be found in industrial wastewater, such as that produced during cork processing []. Advanced oxidation processes, including UV/H2O2 and photo-Fenton systems, have been investigated for their effectiveness in degrading this compound and reducing its environmental impact [].
Q11: What are some important research tools and resources for studying this compound?
A11: Various tools and resources are available to facilitate this compound research, including:
- Databases: Publicly available databases like PubChem and KNApSAcK provide valuable information on the structure, properties, and biological activities of this compound [].
- Software: Molecular docking software, such as Autodock Vina, enables the simulation of this compound interactions with target proteins [].
Q12: What are some key historical milestones in this compound research?
A12: Research on this compound spans several decades. Key milestones include:
- Early Isolation and Identification: this compound was identified in various plant sources, marking the beginning of its exploration [, , ].
- Elucidation of Its Role as an NADPH Oxidase Inhibitor: This discovery sparked significant interest in this compound's therapeutic potential for conditions involving oxidative stress [, , ].
- Investigation of this compound's Metabolic Pathways: Understanding how this compound is metabolized in biological systems is crucial for evaluating its safety and efficacy [].
Q13: How does research on this compound bridge different scientific disciplines?
A13: this compound research exemplifies the interconnectedness of various scientific fields:
- Chemistry: Chemical synthesis, isolation, and characterization techniques are fundamental to studying this compound [, , ].
- Biology: Understanding this compound's interactions with biological systems, including its effects on enzymes, cells, and tissues, is crucial for exploring its therapeutic potential [, , ].
- Pharmacology: Investigating this compound's absorption, distribution, metabolism, and excretion (ADME) profile provides valuable information for its potential use as a drug [].
- Environmental Science: Assessing the environmental fate and potential impact of this compound is essential for its sustainable use [, ].
Disclaimer and Information on In-Vitro Research Products
Please be aware that all articles and product information presented on BenchChem are intended solely for informational purposes. The products available for purchase on BenchChem are specifically designed for in-vitro studies, which are conducted outside of living organisms. In-vitro studies, derived from the Latin term "in glass," involve experiments performed in controlled laboratory settings using cells or tissues. It is important to note that these products are not categorized as medicines or drugs, and they have not received approval from the FDA for the prevention, treatment, or cure of any medical condition, ailment, or disease. We must emphasize that any form of bodily introduction of these products into humans or animals is strictly prohibited by law. It is essential to adhere to these guidelines to ensure compliance with legal and ethical standards in research and experimentation.