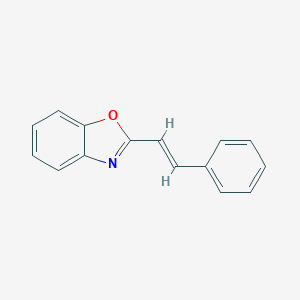
(E)-2-Styrylbenzoxazole
Overview
Description
(E)-2-Styrylbenzoxazole is an organic compound characterized by a benzoxazole ring substituted with a styryl group at the 2-position. This compound is known for its unique photophysical properties, making it a subject of interest in various scientific fields, including materials science and organic chemistry.
Preparation Methods
Synthetic Routes and Reaction Conditions: The synthesis of (E)-2-Styrylbenzoxazole typically involves the condensation of benzoxazole with styrene derivatives. One common method is the Wittig reaction, where a phosphonium ylide reacts with a benzoxazole aldehyde to form the styrylbenzoxazole. The reaction conditions often include the use of a strong base such as sodium hydride or potassium tert-butoxide in an aprotic solvent like tetrahydrofuran.
Industrial Production Methods: Industrial production of this compound may involve similar synthetic routes but on a larger scale. The process is optimized for higher yields and purity, often employing continuous flow reactors and advanced purification techniques such as recrystallization and chromatography.
Chemical Reactions Analysis
Types of Reactions: (E)-2-Styrylbenzoxazole undergoes various chemical reactions, including:
Oxidation: The compound can be oxidized using agents like potassium permanganate or chromium trioxide, leading to the formation of benzoxazole carboxylic acids.
Reduction: Reduction reactions using hydrogenation catalysts such as palladium on carbon can convert the styryl group to an ethyl group.
Common Reagents and Conditions:
Oxidation: Potassium permanganate in acidic or neutral conditions.
Reduction: Hydrogen gas with palladium on carbon catalyst.
Substitution: Halogenating agents like bromine or chlorine in the presence of a Lewis acid catalyst.
Major Products:
Oxidation: Benzoxazole carboxylic acids.
Reduction: 2-Ethylbenzoxazole.
Substitution: Halogenated benzoxazole derivatives.
Scientific Research Applications
(E)-2-Styrylbenzoxazole has a wide range of applications in scientific research:
Chemistry: Used as a fluorescent probe in various analytical techniques due to its strong fluorescence properties.
Biology: Investigated for its potential as a bioimaging agent in cellular studies.
Medicine: Explored for its antimicrobial and anticancer properties.
Industry: Utilized in the development of organic light-emitting diodes (OLEDs) and other optoelectronic devices.
Mechanism of Action
The mechanism of action of (E)-2-Styrylbenzoxazole involves its interaction with specific molecular targets, leading to various biological effects. In bioimaging, the compound’s fluorescence is due to the excitation of electrons in the styryl group, which then emit light upon returning to the ground state. In antimicrobial and anticancer applications, the compound may interact with cellular membranes or DNA, disrupting vital cellular processes.
Comparison with Similar Compounds
2-Styrylbenzothiazole: Similar structure but with a sulfur atom replacing the oxygen in the benzoxazole ring.
2-Styrylbenzimidazole: Contains a nitrogen atom in place of the oxygen in the benzoxazole ring.
2-Styrylquinoline: Features a quinoline ring instead of the benzoxazole ring.
Uniqueness: (E)-2-Styrylbenzoxazole is unique due to its specific photophysical properties, which are influenced by the presence of the oxygen atom in the benzoxazole ring. This gives it distinct fluorescence characteristics compared to its sulfur and nitrogen analogs.
Biological Activity
(E)-2-Styrylbenzoxazole is a compound of significant interest due to its diverse biological activities, particularly in the fields of antimicrobial and anticancer research. This article delves into the biological properties, mechanisms of action, and relevant case studies associated with this compound.
Chemical Structure and Properties
This compound belongs to the benzoxazole family, characterized by a fused benzene and oxazole ring. The presence of the styryl group enhances its lipophilicity, which is crucial for its biological activity. The molecular structure can be represented as follows:
Antimicrobial Properties
Research has shown that this compound exhibits notable antimicrobial activity against various mycobacterial strains, including Mycobacterium tuberculosis and Mycobacterium avium. A study conducted by Wei et al. demonstrated that derivatives of this compound were significantly more effective than standard treatments like isoniazid against these pathogens . The inhibitory concentration (IC50) values indicated a strong correlation between the structure of the compound and its antimicrobial efficacy.
Table 1: Antimycobacterial Activity of this compound Derivatives
Compound | Mycobacterium tuberculosis IC50 (µM) | Mycobacterium avium IC50 (µM) |
---|---|---|
Compound 1 | 1.5 | 0.8 |
Compound 2 | 1.2 | 0.6 |
Compound 3 | 2.0 | 1.5 |
Photosynthesis Inhibition
The compound has also been evaluated for its ability to inhibit photosynthesis in plants, specifically targeting the electron transport chain in chloroplasts. The results indicate that certain derivatives can effectively disrupt photosynthetic electron transport (PET), which is critical for plant energy conversion processes . The study highlighted that the effectiveness of inhibition is influenced by the lipophilicity of the substituents on the benzoxazole ring.
Table 2: PET Inhibition Activity
Compound | Log(1/IC50) (mol/L) | Lipophilicity (log k) |
---|---|---|
Compound A | -3.5 | 3.0 |
Compound B | -4.0 | 2.5 |
Compound C | -3.0 | 3.5 |
The biological activity of this compound can be attributed to several mechanisms:
- Interaction with DNA : Some studies suggest that benzoxazole derivatives can intercalate with DNA, disrupting replication and transcription processes.
- Enzyme Inhibition : The compounds may inhibit key enzymes involved in metabolic pathways, leading to cell death in pathogenic organisms.
- Photosystem II Targeting : The inhibition of PET indicates a specific interaction with components of photosystem II, affecting chlorophyll fluorescence and energy transfer .
Study on Antimycobacterial Activity
In a comprehensive evaluation, a series of twelve derivatives of this compound were synthesized and tested against various strains of mycobacteria. The findings revealed that specific ortho-substituted compounds exhibited superior activity compared to para-substituted ones, emphasizing the importance of molecular configuration in biological efficacy .
Imaging Studies in Alzheimer's Disease
Another significant application of styrylbenzoxazole derivatives is their use in imaging amyloid plaques associated with Alzheimer's disease. A derivative known as BF-168 has shown promise as a selective agent for recognizing amyloid plaques and neurofibrillary tangles in brain tissues . This highlights the potential for these compounds not only as therapeutic agents but also as diagnostic tools.
Properties
IUPAC Name |
2-[(E)-2-phenylethenyl]-1,3-benzoxazole | |
---|---|---|
Source | PubChem | |
URL | https://pubchem.ncbi.nlm.nih.gov | |
Description | Data deposited in or computed by PubChem | |
InChI |
InChI=1S/C15H11NO/c1-2-6-12(7-3-1)10-11-15-16-13-8-4-5-9-14(13)17-15/h1-11H/b11-10+ | |
Source | PubChem | |
URL | https://pubchem.ncbi.nlm.nih.gov | |
Description | Data deposited in or computed by PubChem | |
InChI Key |
GJFNNZBYCMUAHY-ZHACJKMWSA-N | |
Source | PubChem | |
URL | https://pubchem.ncbi.nlm.nih.gov | |
Description | Data deposited in or computed by PubChem | |
Canonical SMILES |
C1=CC=C(C=C1)C=CC2=NC3=CC=CC=C3O2 | |
Source | PubChem | |
URL | https://pubchem.ncbi.nlm.nih.gov | |
Description | Data deposited in or computed by PubChem | |
Isomeric SMILES |
C1=CC=C(C=C1)/C=C/C2=NC3=CC=CC=C3O2 | |
Source | PubChem | |
URL | https://pubchem.ncbi.nlm.nih.gov | |
Description | Data deposited in or computed by PubChem | |
Molecular Formula |
C15H11NO | |
Source | PubChem | |
URL | https://pubchem.ncbi.nlm.nih.gov | |
Description | Data deposited in or computed by PubChem | |
Molecular Weight |
221.25 g/mol | |
Source | PubChem | |
URL | https://pubchem.ncbi.nlm.nih.gov | |
Description | Data deposited in or computed by PubChem | |
Retrosynthesis Analysis
AI-Powered Synthesis Planning: Our tool employs the Template_relevance Pistachio, Template_relevance Bkms_metabolic, Template_relevance Pistachio_ringbreaker, Template_relevance Reaxys, Template_relevance Reaxys_biocatalysis model, leveraging a vast database of chemical reactions to predict feasible synthetic routes.
One-Step Synthesis Focus: Specifically designed for one-step synthesis, it provides concise and direct routes for your target compounds, streamlining the synthesis process.
Accurate Predictions: Utilizing the extensive PISTACHIO, BKMS_METABOLIC, PISTACHIO_RINGBREAKER, REAXYS, REAXYS_BIOCATALYSIS database, our tool offers high-accuracy predictions, reflecting the latest in chemical research and data.
Strategy Settings
Precursor scoring | Relevance Heuristic |
---|---|
Min. plausibility | 0.01 |
Model | Template_relevance |
Template Set | Pistachio/Bkms_metabolic/Pistachio_ringbreaker/Reaxys/Reaxys_biocatalysis |
Top-N result to add to graph | 6 |
Feasible Synthetic Routes
Disclaimer and Information on In-Vitro Research Products
Please be aware that all articles and product information presented on BenchChem are intended solely for informational purposes. The products available for purchase on BenchChem are specifically designed for in-vitro studies, which are conducted outside of living organisms. In-vitro studies, derived from the Latin term "in glass," involve experiments performed in controlled laboratory settings using cells or tissues. It is important to note that these products are not categorized as medicines or drugs, and they have not received approval from the FDA for the prevention, treatment, or cure of any medical condition, ailment, or disease. We must emphasize that any form of bodily introduction of these products into humans or animals is strictly prohibited by law. It is essential to adhere to these guidelines to ensure compliance with legal and ethical standards in research and experimentation.