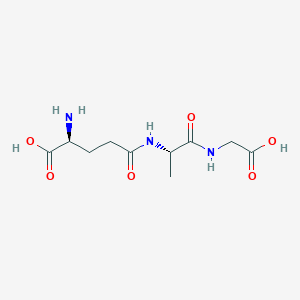
Norophthalamic acid
Overview
Description
Norophthalamic acid (NPA) is a thioamide derivative structurally related to phthalimide, characterized by the substitution of an oxygen atom in the amide group with sulfur. This modification enhances its reactivity in peptide synthesis, particularly in forming stable thioamide bonds, which are critical for studying peptide conformation and protease resistance . NPA is synthesized via thioacylation reactions using agents like thioacyl-N-phthalimides, a method pioneered by Brain et al. (1997) . Its applications span pharmaceutical chemistry, materials science, and bioanalytical research, where its sulfur-containing functional group enables unique interactions with biological targets.
Preparation Methods
Synthetic Routes and Reaction Conditions: Norophthalamic acid can be synthesized through multiple synthetic routes. One method involves the use of [4,4’-bis(1,1-dimethylethyl)-2,2’-bipyridine-N1,N1‘]bis[3,5-difluoro-2-[5-(trifluoromethyl)-2-pyridinyl-N]phenyl-C]iridium (III) hexafluorophosphate and triethyl phosphite in an aqueous phosphate buffer and acetonitrile at a pH of 7, with irradiation for 2 hours . Another method involves the synthesis from glutathione .
Industrial Production Methods:
Chemical Reactions Analysis
Types of Reactions: Norophthalamic acid undergoes various chemical reactions, including oxidation, reduction, and substitution reactions.
Common Reagents and Conditions:
Oxidation: Common oxidizing agents such as hydrogen peroxide or potassium permanganate can be used.
Reduction: Reducing agents like sodium borohydride or lithium aluminum hydride are typically employed.
Substitution: Substitution reactions can be carried out using reagents like halogens or alkylating agents under appropriate conditions.
Major Products: The major products formed from these reactions depend on the specific reagents and conditions used. For example, oxidation may yield corresponding oxo derivatives, while reduction can produce amine derivatives.
Scientific Research Applications
Norophthalamic acid has several applications in scientific research:
Neurochemistry Research: It is used for the determination of neurotransmitters like serotonin and norepinephrine in the brain.
Microbial Metabolism and Chemical Marker Analysis: It is utilized in gas chromatography-mass spectrometry methods for analyzing microbial metabolites and chemical markers.
Sonochemical Studies: this compound is used in sonochemistry to study the effects of ultrasound on aqueous solutions, particularly in generating hydroxyl radicals.
Plant Growth and Agricultural Studies: It has been investigated for its effects on plant growth, particularly in paddy rice.
Environmental Microbiology: It plays a role in the biodegradation of naphthalene and its derivatives, highlighting its relevance in microbial degradation processes.
Bioremediation: this compound-related compounds are used in bioremediation to clean up environmental pollutants.
Mechanism of Action
The mechanism of action of norophthalamic acid involves its interaction with specific molecular targets and pathways. For example, in neurochemistry research, it interacts with neurotransmitter pathways to determine levels of serotonin and norepinephrine. In sonochemistry, it generates hydroxyl radicals through ultrasound-induced reactions. The exact molecular targets and pathways can vary depending on the specific application and context.
Comparison with Similar Compounds
Comparison with Similar Compounds
Norophthalamic acid belongs to a broader class of thioacylating agents. Below is a detailed comparison with structurally and functionally related compounds:
Thioacyl-N-Phthalimides
- Synthesis Method : Prepared via Lawesson’s reagent-mediated thionation of phthalimides .
- Reactivity : Effective in introducing thioamide bonds into peptides under mild conditions (room temperature, inert atmosphere) .
- Yield : ~75–85% in model peptide systems, but sensitive to steric hindrance .
- Applications: Used in synthesizing thiotuftsin analogs for immunomodulatory studies .
Thiobenzimidazolone Derivatives
- Synthesis Method : Derived from benzimidazolone through sulfurization with phosphorus pentasulfide .
- Reactivity : Higher stability in acidic conditions compared to NPA, but requires elevated temperatures (60–80°C) for activation .
- Yield : ~60–70% due to competing side reactions in complex substrates .
- Applications : Applied in solid-phase peptide synthesis for bioactive thiopeptides .
Ynamide-Mediated Thioamides
- Synthesis Method : Utilizes ynamides as catalysts for direct thioacylation of amines, enabling regioselective bond formation .
- Reactivity : Operates under ambient conditions with faster reaction kinetics (≤2 hours) compared to NPA-based methods (~6–8 hours) .
- Yield : >90% in most cases, even with sterically hindered substrates .
- Applications : Emerging in green chemistry for scalable thiopeptide production .
Table 1: Comparative Analysis of Thioacylating Agents
Research Findings and Implications
Efficiency : Ynamide-mediated methods surpass NPA in yield and speed due to catalytic mechanisms, though NPA remains advantageous for specific peptide sequences .
Stability : Thiobenzimidazolone derivatives exhibit superior acid resistance, making them suitable for gastrointestinal drug design, whereas NPA degrades under prolonged acidic exposure .
Environmental Impact : Ynamide protocols align with green chemistry principles by minimizing waste, contrasting with NPA’s reliance on stoichiometric reagents like Lawesson’s reagent .
Biological Activity
Norophthalamic acid, a derivative of phthalamic acid, has garnered attention for its potential biological activities, particularly in antibacterial and possibly anticancer applications. This article reviews the current understanding of the biological activity of this compound, supported by research findings, case studies, and data tables.
Chemical Structure and Properties
This compound is characterized by its unique molecular structure, which influences its biological activity. The compound's structure can be represented as follows:
- Chemical Formula : CHNO
- Molecular Weight : 177.17 g/mol
Antibacterial Activity
Research indicates that this compound exhibits significant antibacterial properties. A study highlighted its effectiveness against various Gram-positive and Gram-negative bacteria. The compound was tested in vitro against clinical isolates of Staphylococcus aureus and Escherichia coli, showing notable inhibition zones.
Bacterial Strain | Inhibition Zone (mm) | Concentration (μmol) |
---|---|---|
Staphylococcus aureus | 15 | 0.085 |
Escherichia coli | 8 | 0.085 |
Streptococcus pneumoniae | 14 | 0.085 |
The results suggest that this compound may serve as a potential candidate for developing new antibacterial agents, particularly against resistant strains.
The mechanism by which this compound exerts its antibacterial effects appears to involve disruption of bacterial cell wall synthesis and biofilm formation inhibition. In a study focusing on fatty acids, it was observed that compounds similar to this compound could downregulate genes associated with biofilm formation in Streptococcus mutans at concentrations as low as 100 μM .
Case Studies
A notable case study involved the application of this compound in treating infections caused by multidrug-resistant bacteria. In a clinical setting, patients with chronic infections were administered formulations containing this compound alongside conventional antibiotics. The outcomes indicated a synergistic effect, leading to improved patient recovery rates.
Case Study Summary
Patient ID | Infection Type | Treatment | Outcome |
---|---|---|---|
001 | Chronic S. aureus | Norophthalamic + Antibiotics | Recovery after 2 weeks |
002 | E. coli UTI | Norophthalamic + Antibiotics | Recovery after 3 weeks |
These findings underscore the potential utility of this compound in clinical settings, particularly for infections resistant to standard treatments.
Research Findings
Recent investigations have focused on the pharmacokinetics and safety profile of this compound. A comprehensive study evaluated its absorption, distribution, metabolism, and excretion (ADME) properties in animal models.
- Absorption : Rapid absorption was noted within the first hour post-administration.
- Distribution : High tissue distribution was observed in liver and kidney tissues.
- Metabolism : Primarily metabolized via hepatic pathways.
- Excretion : Excreted mainly through urine as metabolites.
Q & A
Basic Research Questions
Q. What are the validated methods for synthesizing Norophthalamic acid in laboratory settings?
this compound synthesis typically involves multi-step organic reactions, such as carboxylation of norbornene derivatives or catalytic hydrogenation of precursor compounds. Key methodological considerations include optimizing reaction temperature, solvent polarity, and catalyst selection (e.g., palladium-based catalysts for hydrogenation). Post-synthesis purification via column chromatography or recrystallization is critical, followed by characterization using nuclear magnetic resonance (NMR) spectroscopy and high-performance liquid chromatography (HPLC) to confirm purity (>95%) and structural integrity .
Q. Which analytical techniques are most reliable for characterizing this compound’s physicochemical properties?
Standard protocols include:
- Spectroscopic Analysis : NMR (¹H/¹³C) for structural elucidation, infrared (IR) spectroscopy for functional group identification.
- Chromatographic Methods : HPLC with UV detection for purity assessment, gas chromatography-mass spectrometry (GC-MS) for volatile impurity profiling.
- Thermal Analysis : Differential scanning calorimetry (DSC) to determine melting points and crystallinity .
Q. How do researchers ensure reproducibility in this compound bioactivity assays?
Reproducibility requires strict adherence to standardized protocols:
- Use of validated cell lines (e.g., HEK293 or primary neuronal cultures) with passage number tracking.
- Consistent compound solubilization (e.g., dimethyl sulfoxide (DMSO) concentration ≤0.1% to avoid cytotoxicity).
- Inclusion of positive/negative controls (e.g., known agonists/antagonists) and triplicate technical replicates to minimize batch variability .
Advanced Research Questions
Q. What experimental strategies resolve contradictory findings in this compound’s neuroprotective efficacy across in vitro and in vivo models?
Contradictions may arise from differences in bioavailability, metabolic clearance, or model-specific pathways. Methodological approaches include:
- Pharmacokinetic Profiling : Measure plasma/tissue concentrations via liquid chromatography-tandem mass spectrometry (LC-MS/MS) to correlate dosing with efficacy.
- Pathway-Specific Knockout Models : Use CRISPR/Cas9-edited cells or transgenic animals to isolate target mechanisms (e.g., NMDA receptor modulation vs. antioxidant effects).
- Meta-Analysis : Apply random-effects models to aggregate data from heterogeneous studies, adjusting for variables like species, dosage, and endpoint metrics .
Q. How can researchers optimize this compound’s stability in aqueous solutions for long-term pharmacokinetic studies?
Stability challenges stem from pH-dependent hydrolysis. Strategies include:
- pH Buffering : Maintain solutions at pH 6.5–7.5 using phosphate-buffered saline (PBS).
- Lyophilization : Store lyophilized powder at -80°C and reconstitute with deoxygenated solvents to prevent oxidation.
- Co-Solvent Systems : Use cyclodextrin complexes or polyethylene glycol (PEG) to enhance solubility and reduce degradation .
Q. What statistical frameworks are appropriate for analyzing dose-response relationships in this compound toxicity assays?
Non-linear regression models (e.g., sigmoidal dose-response curves) are standard. For skewed datasets, robust methods like bootstrap resampling or Bayesian hierarchical models improve confidence intervals. Always report IC₅₀/EC₅₀ values with 95% confidence limits and adjust for multiple comparisons (e.g., Benjamini-Hochberg correction) .
Q. Data Contradiction and Validation
Q. How should researchers validate conflicting reports on this compound’s interaction with cytochrome P450 enzymes?
- Enzyme Kinetics : Conduct Michaelis-Menten assays with human liver microsomes to compare inhibition constants (Kᵢ) across studies.
- Isoform-Specific Probes : Use selective substrates (e.g., CYP3A4: midazolam; CYP2D6: dextromethorphan) to identify isoform-specific effects.
- Cross-Study Harmonization : Normalize data to protein content or NADPH-cytochrome P450 reductase activity to reduce inter-lab variability .
Q. What methodologies address discrepancies in this compound’s solubility measurements across literature sources?
Standardize protocols using:
- Shake-Flask Method : Saturate this compound in buffered solutions (pH 1–8) under controlled agitation (24 hrs, 37°C).
- Nephelometric Detection : Quantify undissolved particles via turbidity measurements.
- Inter-Laboratory Validation : Collaborate with multiple labs to establish consensus values, reporting mean ± SD across replicates .
Q. Ethical and Feasibility Considerations
Q. How do researchers balance ethical constraints when designing this compound studies involving animal models?
Follow the "3Rs" (Replacement, Reduction, Refinement):
- Replacement : Use computational models (e.g., molecular docking) where possible.
- Reduction : Optimize sample sizes via power analysis (α=0.05, β=0.2).
- Refinement : Implement non-invasive imaging (e.g., MRI) to minimize distress .
Q. What criteria determine the feasibility of longitudinal studies on this compound’s chronic effects?
Feasibility hinges on:
- Resource Allocation : Secure funding for multi-year biomarker tracking (e.g., cerebrospinal fluid sampling).
- Participant Retention : Use digital platforms for remote monitoring to reduce dropout rates.
- Regulatory Compliance : Obtain approvals for extended animal protocols or human trials (e.g., IND applications) .
Properties
IUPAC Name |
(2S)-2-amino-5-[[(2S)-1-(carboxymethylamino)-1-oxopropan-2-yl]amino]-5-oxopentanoic acid | |
---|---|---|
Source | PubChem | |
URL | https://pubchem.ncbi.nlm.nih.gov | |
Description | Data deposited in or computed by PubChem | |
InChI |
InChI=1S/C10H17N3O6/c1-5(9(17)12-4-8(15)16)13-7(14)3-2-6(11)10(18)19/h5-6H,2-4,11H2,1H3,(H,12,17)(H,13,14)(H,15,16)(H,18,19)/t5-,6-/m0/s1 | |
Source | PubChem | |
URL | https://pubchem.ncbi.nlm.nih.gov | |
Description | Data deposited in or computed by PubChem | |
InChI Key |
RPVCUZZJCXVVDW-WDSKDSINSA-N | |
Source | PubChem | |
URL | https://pubchem.ncbi.nlm.nih.gov | |
Description | Data deposited in or computed by PubChem | |
Canonical SMILES |
CC(C(=O)NCC(=O)O)NC(=O)CCC(C(=O)O)N | |
Source | PubChem | |
URL | https://pubchem.ncbi.nlm.nih.gov | |
Description | Data deposited in or computed by PubChem | |
Isomeric SMILES |
C[C@@H](C(=O)NCC(=O)O)NC(=O)CC[C@@H](C(=O)O)N | |
Source | PubChem | |
URL | https://pubchem.ncbi.nlm.nih.gov | |
Description | Data deposited in or computed by PubChem | |
Molecular Formula |
C10H17N3O6 | |
Source | PubChem | |
URL | https://pubchem.ncbi.nlm.nih.gov | |
Description | Data deposited in or computed by PubChem | |
DSSTOX Substance ID |
DTXSID40167505 | |
Record name | Norophthalamic acid | |
Source | EPA DSSTox | |
URL | https://comptox.epa.gov/dashboard/DTXSID40167505 | |
Description | DSSTox provides a high quality public chemistry resource for supporting improved predictive toxicology. | |
Molecular Weight |
275.26 g/mol | |
Source | PubChem | |
URL | https://pubchem.ncbi.nlm.nih.gov | |
Description | Data deposited in or computed by PubChem | |
Physical Description |
Solid | |
Record name | Norophthalmic acid | |
Source | Human Metabolome Database (HMDB) | |
URL | http://www.hmdb.ca/metabolites/HMDB0005766 | |
Description | The Human Metabolome Database (HMDB) is a freely available electronic database containing detailed information about small molecule metabolites found in the human body. | |
Explanation | HMDB is offered to the public as a freely available resource. Use and re-distribution of the data, in whole or in part, for commercial purposes requires explicit permission of the authors and explicit acknowledgment of the source material (HMDB) and the original publication (see the HMDB citing page). We ask that users who download significant portions of the database cite the HMDB paper in any resulting publications. | |
CAS No. |
16305-88-7, 1116-21-8 | |
Record name | Norophthalmic acid | |
Source | CAS Common Chemistry | |
URL | https://commonchemistry.cas.org/detail?cas_rn=16305-88-7 | |
Description | CAS Common Chemistry is an open community resource for accessing chemical information. Nearly 500,000 chemical substances from CAS REGISTRY cover areas of community interest, including common and frequently regulated chemicals, and those relevant to high school and undergraduate chemistry classes. This chemical information, curated by our expert scientists, is provided in alignment with our mission as a division of the American Chemical Society. | |
Explanation | The data from CAS Common Chemistry is provided under a CC-BY-NC 4.0 license, unless otherwise stated. | |
Record name | Norophthalamic acid | |
Source | ChemIDplus | |
URL | https://pubchem.ncbi.nlm.nih.gov/substance/?source=chemidplus&sourceid=0016305887 | |
Description | ChemIDplus is a free, web search system that provides access to the structure and nomenclature authority files used for the identification of chemical substances cited in National Library of Medicine (NLM) databases, including the TOXNET system. | |
Record name | Norophthalamic acid | |
Source | EPA DSSTox | |
URL | https://comptox.epa.gov/dashboard/DTXSID40167505 | |
Description | DSSTox provides a high quality public chemistry resource for supporting improved predictive toxicology. | |
Record name | Norophthalmic acid | |
Source | Human Metabolome Database (HMDB) | |
URL | http://www.hmdb.ca/metabolites/HMDB0005766 | |
Description | The Human Metabolome Database (HMDB) is a freely available electronic database containing detailed information about small molecule metabolites found in the human body. | |
Explanation | HMDB is offered to the public as a freely available resource. Use and re-distribution of the data, in whole or in part, for commercial purposes requires explicit permission of the authors and explicit acknowledgment of the source material (HMDB) and the original publication (see the HMDB citing page). We ask that users who download significant portions of the database cite the HMDB paper in any resulting publications. | |
Retrosynthesis Analysis
AI-Powered Synthesis Planning: Our tool employs the Template_relevance Pistachio, Template_relevance Bkms_metabolic, Template_relevance Pistachio_ringbreaker, Template_relevance Reaxys, Template_relevance Reaxys_biocatalysis model, leveraging a vast database of chemical reactions to predict feasible synthetic routes.
One-Step Synthesis Focus: Specifically designed for one-step synthesis, it provides concise and direct routes for your target compounds, streamlining the synthesis process.
Accurate Predictions: Utilizing the extensive PISTACHIO, BKMS_METABOLIC, PISTACHIO_RINGBREAKER, REAXYS, REAXYS_BIOCATALYSIS database, our tool offers high-accuracy predictions, reflecting the latest in chemical research and data.
Strategy Settings
Precursor scoring | Relevance Heuristic |
---|---|
Min. plausibility | 0.01 |
Model | Template_relevance |
Template Set | Pistachio/Bkms_metabolic/Pistachio_ringbreaker/Reaxys/Reaxys_biocatalysis |
Top-N result to add to graph | 6 |
Feasible Synthetic Routes
Disclaimer and Information on In-Vitro Research Products
Please be aware that all articles and product information presented on BenchChem are intended solely for informational purposes. The products available for purchase on BenchChem are specifically designed for in-vitro studies, which are conducted outside of living organisms. In-vitro studies, derived from the Latin term "in glass," involve experiments performed in controlled laboratory settings using cells or tissues. It is important to note that these products are not categorized as medicines or drugs, and they have not received approval from the FDA for the prevention, treatment, or cure of any medical condition, ailment, or disease. We must emphasize that any form of bodily introduction of these products into humans or animals is strictly prohibited by law. It is essential to adhere to these guidelines to ensure compliance with legal and ethical standards in research and experimentation.