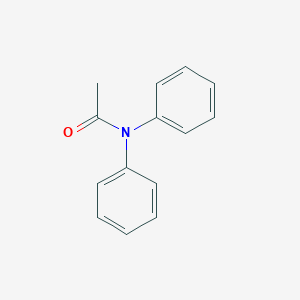
N,N-Diphenylacetamide
Overview
Description
CH₃CON(C₆H₅)₂ . It appears as a white crystalline powder and is known for its ability to sublimate without decomposition. This compound is soluble in ethanol and ether but only slightly soluble in water .
Preparation Methods
Synthetic Routes and Reaction Conditions: N,N-Diphenylacetamide can be synthesized through the reaction of acetic anhydride with aniline in the presence of a catalyst. The reaction typically proceeds as follows: [ \text{CH}_3\text{CO}_2\text{O} + 2 \text{C}_6\text{H}_5\text{NH}_2 \rightarrow \text{CH}_3\text{CON(C}_6\text{H}_5\text{)}_2 + \text{CH}_3\text{COOH} ]
Industrial Production Methods: In industrial settings, the production of this compound often involves the use of acetic anhydride and aniline under controlled temperature and pressure conditions to ensure high yield and purity. The reaction is typically carried out in a solvent such as toluene or xylene to facilitate the reaction and subsequent purification steps .
Chemical Reactions Analysis
Types of Reactions: N,N-Diphenylacetamide undergoes various chemical reactions, including:
Oxidation: It can be oxidized to form this compound oxide.
Reduction: It can be reduced to form N,N-diphenylethylamine.
Substitution: It can undergo substitution reactions where the acetamide group is replaced by other functional groups.
Common Reagents and Conditions:
Oxidation: Common oxidizing agents include potassium permanganate and chromium trioxide.
Reduction: Common reducing agents include lithium aluminum hydride and sodium borohydride.
Substitution: Reagents such as halogens and alkylating agents are used under various conditions.
Major Products:
Oxidation: this compound oxide.
Reduction: N,N-Diphenylethylamine.
Substitution: Various substituted acetamides depending on the reagents used
Scientific Research Applications
N,N-Diphenylacetamide has a wide range of applications in scientific research:
Chemistry: It is used as a ligand in coordination chemistry to form complexes with metals.
Biology: It is used in the study of enzyme inhibition and protein-ligand interactions.
Industry: It is used as an intermediate in the synthesis of dyes, pigments, and other organic compounds
Mechanism of Action
N,N-Diphenylacetamide exerts its effects primarily through its ability to act as a ligand, forming complexes with metal ions. This interaction can influence various biochemical pathways, including enzyme activity and protein function. The compound’s N,N-disubstituted amide group allows it to act as both a mono- and bidentate ligand, generating four-membered chelate rings .
Comparison with Similar Compounds
- N,N-Diethylacetamide
- N,N-Dimethylacetamide
- N,N′-Diphenylbenzidine
Comparison: N,N-Diphenylacetamide is unique due to its ability to form stable complexes with metal ions, which is not as pronounced in similar compounds like N,N-Diethylacetamide and N,N-Dimethylacetamide. Additionally, its higher molecular weight and specific structural features make it more suitable for certain applications in coordination chemistry and drug development .
Properties
IUPAC Name |
N,N-diphenylacetamide | |
---|---|---|
Source | PubChem | |
URL | https://pubchem.ncbi.nlm.nih.gov | |
Description | Data deposited in or computed by PubChem | |
InChI |
InChI=1S/C14H13NO/c1-12(16)15(13-8-4-2-5-9-13)14-10-6-3-7-11-14/h2-11H,1H3 | |
Source | PubChem | |
URL | https://pubchem.ncbi.nlm.nih.gov | |
Description | Data deposited in or computed by PubChem | |
InChI Key |
DKLYDESVXZKCFI-UHFFFAOYSA-N | |
Source | PubChem | |
URL | https://pubchem.ncbi.nlm.nih.gov | |
Description | Data deposited in or computed by PubChem | |
Canonical SMILES |
CC(=O)N(C1=CC=CC=C1)C2=CC=CC=C2 | |
Source | PubChem | |
URL | https://pubchem.ncbi.nlm.nih.gov | |
Description | Data deposited in or computed by PubChem | |
Molecular Formula |
C14H13NO | |
Source | PubChem | |
URL | https://pubchem.ncbi.nlm.nih.gov | |
Description | Data deposited in or computed by PubChem | |
DSSTOX Substance ID |
DTXSID8060165 | |
Record name | Acetamide, N,N-diphenyl- | |
Source | EPA DSSTox | |
URL | https://comptox.epa.gov/dashboard/DTXSID8060165 | |
Description | DSSTox provides a high quality public chemistry resource for supporting improved predictive toxicology. | |
Molecular Weight |
211.26 g/mol | |
Source | PubChem | |
URL | https://pubchem.ncbi.nlm.nih.gov | |
Description | Data deposited in or computed by PubChem | |
CAS No. |
519-87-9 | |
Record name | N,N-Diphenylacetamide | |
Source | CAS Common Chemistry | |
URL | https://commonchemistry.cas.org/detail?cas_rn=519-87-9 | |
Description | CAS Common Chemistry is an open community resource for accessing chemical information. Nearly 500,000 chemical substances from CAS REGISTRY cover areas of community interest, including common and frequently regulated chemicals, and those relevant to high school and undergraduate chemistry classes. This chemical information, curated by our expert scientists, is provided in alignment with our mission as a division of the American Chemical Society. | |
Explanation | The data from CAS Common Chemistry is provided under a CC-BY-NC 4.0 license, unless otherwise stated. | |
Record name | Diphenylacetamide | |
Source | ChemIDplus | |
URL | https://pubchem.ncbi.nlm.nih.gov/substance/?source=chemidplus&sourceid=0000519879 | |
Description | ChemIDplus is a free, web search system that provides access to the structure and nomenclature authority files used for the identification of chemical substances cited in National Library of Medicine (NLM) databases, including the TOXNET system. | |
Record name | N,N-Diphenylacetamide | |
Source | DTP/NCI | |
URL | https://dtp.cancer.gov/dtpstandard/servlet/dwindex?searchtype=NSC&outputformat=html&searchlist=3133 | |
Description | The NCI Development Therapeutics Program (DTP) provides services and resources to the academic and private-sector research communities worldwide to facilitate the discovery and development of new cancer therapeutic agents. | |
Explanation | Unless otherwise indicated, all text within NCI products is free of copyright and may be reused without our permission. Credit the National Cancer Institute as the source. | |
Record name | Acetamide, N,N-diphenyl- | |
Source | EPA Chemicals under the TSCA | |
URL | https://www.epa.gov/chemicals-under-tsca | |
Description | EPA Chemicals under the Toxic Substances Control Act (TSCA) collection contains information on chemicals and their regulations under TSCA, including non-confidential content from the TSCA Chemical Substance Inventory and Chemical Data Reporting. | |
Record name | Acetamide, N,N-diphenyl- | |
Source | EPA DSSTox | |
URL | https://comptox.epa.gov/dashboard/DTXSID8060165 | |
Description | DSSTox provides a high quality public chemistry resource for supporting improved predictive toxicology. | |
Record name | N,N-diphenylacetamide | |
Source | European Chemicals Agency (ECHA) | |
URL | https://echa.europa.eu/substance-information/-/substanceinfo/100.007.526 | |
Description | The European Chemicals Agency (ECHA) is an agency of the European Union which is the driving force among regulatory authorities in implementing the EU's groundbreaking chemicals legislation for the benefit of human health and the environment as well as for innovation and competitiveness. | |
Explanation | Use of the information, documents and data from the ECHA website is subject to the terms and conditions of this Legal Notice, and subject to other binding limitations provided for under applicable law, the information, documents and data made available on the ECHA website may be reproduced, distributed and/or used, totally or in part, for non-commercial purposes provided that ECHA is acknowledged as the source: "Source: European Chemicals Agency, http://echa.europa.eu/". Such acknowledgement must be included in each copy of the material. ECHA permits and encourages organisations and individuals to create links to the ECHA website under the following cumulative conditions: Links can only be made to webpages that provide a link to the Legal Notice page. | |
Record name | DIPHENYLACETAMIDE | |
Source | FDA Global Substance Registration System (GSRS) | |
URL | https://gsrs.ncats.nih.gov/ginas/app/beta/substances/35M55LM81K | |
Description | The FDA Global Substance Registration System (GSRS) enables the efficient and accurate exchange of information on what substances are in regulated products. Instead of relying on names, which vary across regulatory domains, countries, and regions, the GSRS knowledge base makes it possible for substances to be defined by standardized, scientific descriptions. | |
Explanation | Unless otherwise noted, the contents of the FDA website (www.fda.gov), both text and graphics, are not copyrighted. They are in the public domain and may be republished, reprinted and otherwise used freely by anyone without the need to obtain permission from FDA. Credit to the U.S. Food and Drug Administration as the source is appreciated but not required. | |
Retrosynthesis Analysis
AI-Powered Synthesis Planning: Our tool employs the Template_relevance Pistachio, Template_relevance Bkms_metabolic, Template_relevance Pistachio_ringbreaker, Template_relevance Reaxys, Template_relevance Reaxys_biocatalysis model, leveraging a vast database of chemical reactions to predict feasible synthetic routes.
One-Step Synthesis Focus: Specifically designed for one-step synthesis, it provides concise and direct routes for your target compounds, streamlining the synthesis process.
Accurate Predictions: Utilizing the extensive PISTACHIO, BKMS_METABOLIC, PISTACHIO_RINGBREAKER, REAXYS, REAXYS_BIOCATALYSIS database, our tool offers high-accuracy predictions, reflecting the latest in chemical research and data.
Strategy Settings
Precursor scoring | Relevance Heuristic |
---|---|
Min. plausibility | 0.01 |
Model | Template_relevance |
Template Set | Pistachio/Bkms_metabolic/Pistachio_ringbreaker/Reaxys/Reaxys_biocatalysis |
Top-N result to add to graph | 6 |
Feasible Synthetic Routes
Disclaimer and Information on In-Vitro Research Products
Please be aware that all articles and product information presented on BenchChem are intended solely for informational purposes. The products available for purchase on BenchChem are specifically designed for in-vitro studies, which are conducted outside of living organisms. In-vitro studies, derived from the Latin term "in glass," involve experiments performed in controlled laboratory settings using cells or tissues. It is important to note that these products are not categorized as medicines or drugs, and they have not received approval from the FDA for the prevention, treatment, or cure of any medical condition, ailment, or disease. We must emphasize that any form of bodily introduction of these products into humans or animals is strictly prohibited by law. It is essential to adhere to these guidelines to ensure compliance with legal and ethical standards in research and experimentation.