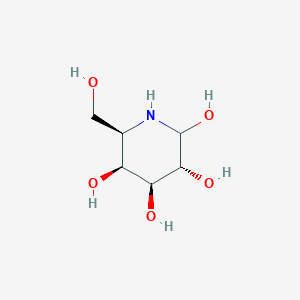
Galactostatin
Overview
Description
Galactostatin is a β-galactosidase inhibitor isolated from the bacterium Streptomyces lydicus PA-5726. Its structure is identified as 5-amino-5-deoxygalactopyranose, where the ring oxygen of galactose is replaced by nitrogen . This compound has shown significant inhibitory activity against β-galactosidase enzymes, making it a valuable tool in biochemical research and potential therapeutic applications .
Preparation Methods
Synthetic Routes and Reaction Conditions
Galactostatin can be synthesized through a stereoselective total synthesis starting from N-tert-butoxycarbonyl-2,3-isopropylidene L-serine methyl ester. The synthesis involves several steps, including the formation of thiazole intermediates that serve as protected aldehydes . The overall yield of this synthesis is approximately 21%.
Industrial Production Methods
Industrial production of this compound involves fermentation using Streptomyces lydicus PA-5726. The compound is isolated by adsorption on Dowex-50W × 8 (H+), crystallization from the bisulfite adduct, development on Dowex-2 × 8 (OH−), and precipitation with ethanol . Optimization of the fermentation medium can significantly increase the yield of this compound.
Chemical Reactions Analysis
Types of Reactions
Galactostatin undergoes various chemical reactions, including oxidation and reduction. The oxidation product is 5-amino-5-deoxygalactonic-δ-lactam (this compound-lactam), and the reduction product is 5-amino-1,5-dideoxygalactopyranose (1-deoxythis compound) .
Common Reagents and Conditions
Oxidation: Common oxidizing agents such as potassium permanganate or chromium trioxide can be used.
Reduction: Reducing agents like sodium borohydride or lithium aluminum hydride are typically employed.
Major Products
Oxidation: 5-amino-5-deoxygalactonic-δ-lactam
Reduction: 5-amino-1,5-dideoxygalactopyranose
Scientific Research Applications
Galactostatin has several scientific research applications:
Biochemistry: Used as a tool to study the inhibition of β-galactosidase enzymes.
Medicine: Potential therapeutic applications in treating diseases related to β-galactosidase activity.
Industrial: Used in the dairy industry to study lactose hydrolysis and the production of lactose-free products.
Mechanism of Action
Galactostatin inhibits β-galactosidase by binding to the active site of the enzyme, preventing the hydrolysis of β-galactosides. This inhibition is competitive, with this compound and its derivatives showing high affinity for the enzyme . The molecular target is the β-galactosidase enzyme, and the pathway involves the disruption of the enzyme’s catalytic activity.
Comparison with Similar Compounds
Similar Compounds
- Galactostatin-lactam
- 1-Deoxythis compound
Uniqueness
This compound is unique due to its strong inhibitory activity and high affinity for β-galactosidase. Its derivatives, such as this compound-lactam and 1-deoxythis compound, also exhibit inhibitory properties but with varying degrees of effectiveness . The structural modification of replacing the ring oxygen with nitrogen in this compound is a distinctive feature that contributes to its potent inhibitory activity .
Biological Activity
Galactostatin is a notable β-galactosidase inhibitor derived from the fermentation broth of Streptomyces lydicus PA-5726. This compound has garnered attention for its potential applications in biochemistry and pharmacology due to its ability to inhibit β-galactosidase enzymes, which play critical roles in various biological processes, including lactose metabolism and cell signaling.
Chemical Structure and Derivatives
The chemical structure of this compound is identified as 5-amino-5-deoxygalactopyranose, where the ring oxygen of galactose is replaced by nitrogen. Its derivatives include:
- This compound-lactam : Formed through oxidation, this derivative exhibits altered biological properties.
- 1-Deoxythis compound : Resulting from reduction, this compound also retains inhibitory functions against β-galactosidases.
These modifications can influence the potency and specificity of this compound's inhibitory effects on various enzymes.
This compound demonstrates a strong inhibitory activity against β-galactosidases, which are enzymes responsible for hydrolyzing β-galactosides into monosaccharides. The inhibition mechanism primarily involves competitive inhibition, where this compound binds to the active site of the enzyme, preventing substrate access. This was evidenced by studies showing IC50 values ranging from 0.59 µM to 1.18 µM against specific β-galactosidases like Nf-LacZ and WspA1 .
Inhibition Studies
Research indicates that this compound exhibits significant inhibitory activity in both acidic and neutral environments. It has been shown to inhibit several β-galactosidases effectively, making it a valuable tool in studying enzyme kinetics and carbohydrate metabolism . The low acute toxicity (LD50 of 1016 mg/kg in mice) further supports its potential for therapeutic applications .
Case Studies
- Inhibition of Lactose Metabolism : In studies involving lactose-intolerant models, this compound's ability to inhibit β-galactosidase has been explored as a means to manage lactose metabolism, potentially offering a dietary supplement for individuals with lactose intolerance.
- Cancer Research : this compound's role in modulating glycosylation patterns in cancer cells has been investigated, with implications for tumor progression and metastasis due to altered cell signaling pathways influenced by glycan structures.
Summary of Research Findings
Properties
IUPAC Name |
(3R,4S,5S,6R)-6-(hydroxymethyl)piperidine-2,3,4,5-tetrol | |
---|---|---|
Source | PubChem | |
URL | https://pubchem.ncbi.nlm.nih.gov | |
Description | Data deposited in or computed by PubChem | |
InChI |
InChI=1S/C6H13NO5/c8-1-2-3(9)4(10)5(11)6(12)7-2/h2-12H,1H2/t2-,3+,4+,5-,6?/m1/s1 | |
Source | PubChem | |
URL | https://pubchem.ncbi.nlm.nih.gov | |
Description | Data deposited in or computed by PubChem | |
InChI Key |
BGMYHTUCJVZIRP-SVZMEOIVSA-N | |
Source | PubChem | |
URL | https://pubchem.ncbi.nlm.nih.gov | |
Description | Data deposited in or computed by PubChem | |
Canonical SMILES |
C(C1C(C(C(C(N1)O)O)O)O)O | |
Source | PubChem | |
URL | https://pubchem.ncbi.nlm.nih.gov | |
Description | Data deposited in or computed by PubChem | |
Isomeric SMILES |
C([C@@H]1[C@@H]([C@@H]([C@H](C(N1)O)O)O)O)O | |
Source | PubChem | |
URL | https://pubchem.ncbi.nlm.nih.gov | |
Description | Data deposited in or computed by PubChem | |
Molecular Formula |
C6H13NO5 | |
Source | PubChem | |
URL | https://pubchem.ncbi.nlm.nih.gov | |
Description | Data deposited in or computed by PubChem | |
DSSTOX Substance ID |
DTXSID50920542 | |
Record name | 6-(Hydroxymethyl)piperidine-2,3,4,5-tetrol | |
Source | EPA DSSTox | |
URL | https://comptox.epa.gov/dashboard/DTXSID50920542 | |
Description | DSSTox provides a high quality public chemistry resource for supporting improved predictive toxicology. | |
Molecular Weight |
179.17 g/mol | |
Source | PubChem | |
URL | https://pubchem.ncbi.nlm.nih.gov | |
Description | Data deposited in or computed by PubChem | |
CAS No. |
107537-94-0 | |
Record name | 5-Amino-5-deoxygalactopyranose | |
Source | ChemIDplus | |
URL | https://pubchem.ncbi.nlm.nih.gov/substance/?source=chemidplus&sourceid=0107537940 | |
Description | ChemIDplus is a free, web search system that provides access to the structure and nomenclature authority files used for the identification of chemical substances cited in National Library of Medicine (NLM) databases, including the TOXNET system. | |
Record name | 6-(Hydroxymethyl)piperidine-2,3,4,5-tetrol | |
Source | EPA DSSTox | |
URL | https://comptox.epa.gov/dashboard/DTXSID50920542 | |
Description | DSSTox provides a high quality public chemistry resource for supporting improved predictive toxicology. | |
Retrosynthesis Analysis
AI-Powered Synthesis Planning: Our tool employs the Template_relevance Pistachio, Template_relevance Bkms_metabolic, Template_relevance Pistachio_ringbreaker, Template_relevance Reaxys, Template_relevance Reaxys_biocatalysis model, leveraging a vast database of chemical reactions to predict feasible synthetic routes.
One-Step Synthesis Focus: Specifically designed for one-step synthesis, it provides concise and direct routes for your target compounds, streamlining the synthesis process.
Accurate Predictions: Utilizing the extensive PISTACHIO, BKMS_METABOLIC, PISTACHIO_RINGBREAKER, REAXYS, REAXYS_BIOCATALYSIS database, our tool offers high-accuracy predictions, reflecting the latest in chemical research and data.
Strategy Settings
Precursor scoring | Relevance Heuristic |
---|---|
Min. plausibility | 0.01 |
Model | Template_relevance |
Template Set | Pistachio/Bkms_metabolic/Pistachio_ringbreaker/Reaxys/Reaxys_biocatalysis |
Top-N result to add to graph | 6 |
Feasible Synthetic Routes
Disclaimer and Information on In-Vitro Research Products
Please be aware that all articles and product information presented on BenchChem are intended solely for informational purposes. The products available for purchase on BenchChem are specifically designed for in-vitro studies, which are conducted outside of living organisms. In-vitro studies, derived from the Latin term "in glass," involve experiments performed in controlled laboratory settings using cells or tissues. It is important to note that these products are not categorized as medicines or drugs, and they have not received approval from the FDA for the prevention, treatment, or cure of any medical condition, ailment, or disease. We must emphasize that any form of bodily introduction of these products into humans or animals is strictly prohibited by law. It is essential to adhere to these guidelines to ensure compliance with legal and ethical standards in research and experimentation.