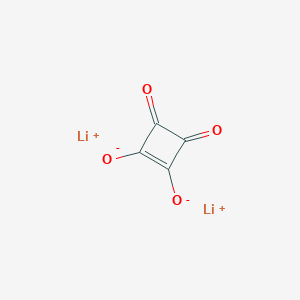
Lithium squarate
Overview
Description
Lithium squarate (dithis compound, Li₂C₄O₄) is an organolithium compound derived from squaric acid (C₄O₄H₂). It consists of a planar squarate anion (C₄O₄²⁻) coordinated with two lithium ions. This compound has garnered attention in energy storage research, particularly as a sacrificial lithium salt for pre-lithiation of anodes in lithium-ion batteries (LIBs) . Its crystal structure, confirmed by X-ray diffraction (XRD), aligns with the dithis compound phase (PDF #04-012-0316), where lithium ions are bonded within the squarate framework .
Key properties of this compound include:
- High theoretical lithium content: Li₂C₄O₄ delivers a specific capacity of up to 3,845 mAh g⁻¹ when fully decomposed, assuming complete expulsion of gaseous byproducts .
- Insulating nature: Bare Li₂C₄O₄ is an electrical insulator, necessitating composite formulations (e.g., with carbon nanotubes) to enhance conductivity .
Preparation Methods
The synthetic route for WAY-100635 maleate salt involves the reaction of N-[2-[4-(2-Methoxyphenyl)-1-piperazinyl]ethyl]-N-2-pyridinylcyclohexanecarboxamide with maleic acid . The reaction conditions typically involve the use of solvents such as dimethyl sulfoxide (DMSO) and water . Industrial production methods for this compound are not widely documented, but the synthesis generally follows similar laboratory procedures with scaled-up reaction conditions .
Chemical Reactions Analysis
WAY-100635 maleate salt undergoes various types of chemical reactions, including:
Oxidation: This reaction can be carried out using oxidizing agents such as hydrogen peroxide or potassium permanganate.
Reduction: Reduction reactions may involve reagents like lithium aluminum hydride or sodium borohydride.
Substitution: Common reagents for substitution reactions include halogens and nucleophiles like hydroxide ions.
The major products formed from these reactions depend on the specific reagents and conditions used. For example, oxidation may yield hydroxylated derivatives, while reduction can produce amine derivatives .
Scientific Research Applications
Pre-Lithiation Additive in Lithium-Ion Batteries
One of the most prominent applications of lithium squarate is as a pre-lithiation additive in lithium-ion batteries. Research conducted by the MEET Battery Research Center at the University of Münster has demonstrated that this compound can significantly prolong the cycle life of silicon-based LIBs.
- Cycle Life Improvement : The addition of this compound increases the cycle life of batteries containing silicon anodes by providing excess lithium ions during the initial charging cycle. This compensates for active lithium losses due to solid electrolyte interphase (SEI) formation at the anode surface .
- Air Stability : Unlike many other pre-lithiation compounds, this compound exhibits air stability, which is crucial for practical applications in battery manufacturing .
- Gas Development and Porosity : While beneficial, the decomposition of this compound during battery operation can lead to gas development and increased porosity, which presents challenges that require further investigation .
Potential for Commercialization
The findings suggest that this compound's characteristics make it a viable candidate for commercial applications in high-energy-density LIBs. The research highlights its compatibility with standard cathode processing protocols, eliminating the need for additional production steps .
Study on NMC622-Silicon/Graphite Full Cells
A comprehensive study published in "Advanced Science" explored the use of this compound as a pre-lithiation additive for NMC622-based positive electrodes paired with silicon/graphite anodes. Key findings include:
- Linear Relationship : The cycle life improvement was found to be linear with respect to the initial amount of this compound added, demonstrating a direct correlation between additive concentration and battery performance .
- Electrode Morphology Changes : Post-mortem analysis revealed significant compositional changes in the cathode electrolyte interphase (CEI), indicating that this compound not only enhances performance but also alters the electrochemical environment within the battery .
Comparative Data Table
Property | This compound | Other Pre-Lithiation Additives |
---|---|---|
Stability | Air-stable | Often unstable |
Compatibility | High | Varies |
Cycle Life Improvement | Significant | Varies |
Gas Development | Moderate | High |
Porosity Increase | Moderate | High |
Mechanism of Action
WAY-100635 maleate salt exerts its effects by selectively binding to and inhibiting 5-HT1A serotonin receptors . This inhibition prevents the activation of these receptors by serotonin, thereby modulating serotonin signaling pathways. The compound’s ability to inhibit brexpiprazole further highlights its role in regulating serotonin-dopamine activity .
Comparison with Similar Compounds
Comparison with Similar Lithium Salts
Lithium Oxalate (Li₂C₂O₄)
- Structure and function : A dicarboxylate salt with a linear C₂O₄²⁻ anion. Like Li₂C₄O₄, it serves as a sacrificial lithium source.
- Performance :
Lithium Acetate (CH₃COOLi)
- Mechanism : Undergoes Kolbe electrolysis to release lithium via irreversible decarboxylation .
- Advantages :
- Drawbacks: Limited lithium content and slower decomposition kinetics.
Dilithium Organics: Li₂DHBN and Li₂DHBA
- Li₂DHBN (3,4-dihydroxybenzonitrile dilithium) and Li₂DHBA (3,4-dihydroxybenzoic acid dilithium):
Data Table: Key Parameters of Lithium Salts
Research Findings and Challenges
- Gas Management : Both Li₂C₄O₄ and Li₂C₂O₄ require advanced cell designs to expel gases (e.g., functional separators) to prevent electrode damage .
- Composite Strategies : Li₂C₄O₄–CNT composites improve conductivity by 3–4 orders of magnitude, enabling practical use .
- Capacity Trade-offs : While Li₂C₄O₄ offers the highest capacity, its gas-related risks make Li₂DHBN/Li₂DHBA preferable for long-cycle applications .
Biological Activity
Lithium squarate, chemically represented as LiCO, has garnered attention for its potential applications in various fields, particularly in battery technology and medicinal chemistry. This article explores the biological activity of this compound, highlighting its mechanisms of action, applications, and relevant research findings.
Chemical Structure and Properties
This compound is a lithium salt derived from squaric acid. Its molecular structure consists of two lithium ions coordinated with a squarate anion, which contains four carbon atoms and four oxygen atoms arranged in a cyclic manner. This unique structure contributes to its stability and functionality in various applications.
Mechanisms of Biological Activity
Lithium itself is known for its mood-stabilizing properties, primarily used in the treatment of bipolar disorder. This compound's biological activity is hypothesized to stem from its ability to release lithium ions in physiological conditions, which may exert neuroprotective effects. The potential mechanisms include:
- Neuroprotection : Lithium ions can modulate neurotransmitter levels and enhance neurotrophic factors, promoting neuronal survival.
- Antioxidant Activity : this compound may exhibit antioxidant properties, reducing oxidative stress in neural tissues.
- Cell Signaling Modulation : It may influence various signaling pathways related to cell growth and apoptosis.
Research Findings
Recent studies have investigated the efficacy of this compound in different contexts:
-
Pre-Lithiation Additive in Lithium-Ion Batteries :
- This compound has been demonstrated as an effective pre-lithiation additive for silicon-based anodes in lithium-ion batteries. It enhances the cycle life by providing additional lithium ions during the initial charge cycle, leading to improved capacity retention over time. A study showed that cells with this compound exhibited a remarkable 97.2% capacity retention after 700 cycles compared to 80% for control cells .
-
Potential Therapeutic Applications :
- The neuroprotective effects of lithium compounds have been explored extensively. Research indicates that this compound could serve as a novel therapeutic agent for neurodegenerative diseases due to its ability to stabilize mood and potentially enhance cognitive function through neuroprotective mechanisms .
Table 1: Comparison of this compound with Other Lithium Compounds
Compound | Biological Activity | Application Area | Stability |
---|---|---|---|
Lithium Carbonate | Mood stabilization | Psychiatry | High |
This compound | Neuroprotection | Battery technology, Psychiatry | Moderate |
Lithium Orotate | Neuroprotective effects | Alternative medicine | Low |
Case Study 1: this compound as a Pre-Lithiation Additive
In a study conducted at the University of Münster, researchers found that incorporating this compound into silicon-based anodes significantly improved the electrochemical performance of lithium-ion batteries. The additive was shown to mitigate capacity loss due to volume changes during cycling, demonstrating its dual role as both a stabilizer and a source of active lithium .
Case Study 2: Neuroprotective Effects
A preliminary investigation into the neuroprotective properties of this compound highlighted its potential use in treating conditions like Alzheimer's disease. The study suggested that this compound could enhance neuronal resilience against stressors by modulating key signaling pathways associated with cell survival .
Q & A
Basic Research Questions
Q. What are the established protocols for synthesizing lithium squarate with high purity?
- Methodological Answer : this compound synthesis typically involves reacting squaric acid (3,4-dihydroxycyclobut-3-ene-1,2-dione) with lithium hydroxide or carbonate in aqueous or alcoholic solvents. Key steps include stoichiometric control (e.g., 1:2 molar ratio of squaric acid to LiOH), reflux conditions (70–90°C for 4–6 hours), and purification via recrystallization from ethanol/water mixtures. Purity is validated using Fourier-transform infrared spectroscopy (FTIR) to confirm characteristic C=O (∼1770 cm⁻¹) and C-O (∼1300 cm⁻¹) stretches, alongside X-ray diffraction (XRD) to verify crystallinity .
Q. How do researchers characterize the thermal stability of this compound?
- Methodological Answer : Thermogravimetric analysis (TGA) and differential thermal analysis (DTA) are standard. For example, TGA under nitrogen flow (heating rate: 10°C/min) reveals decomposition stages: loss of coordinated water (∼100–150°C), followed by squarate anion breakdown (∼250–400°C). DTA endothermic peaks correlate with structural transitions. Complementary data from evolved gas analysis (EGA) via mass spectrometry can identify volatile decomposition products (e.g., CO₂, H₂O) .
Q. What spectral markers in IR and Raman spectroscopy distinguish this compound from its analogs?
- Methodological Answer : Key IR bands include ν(OH) stretches (∼3600 cm⁻¹) for coordinated water and ν(C=O) at ∼1770 cm⁻¹. Raman spectroscopy highlights symmetric squarate ring vibrations (∼1600 cm⁻¹). Discrepancies in band splitting or shifts (e.g., ν(NH₂) at 3329–3380 cm⁻¹ in Co(II)-squarate complexes) can indicate metal-ligand interactions or hydrogen-bonding networks. Cross-referencing with crystal structure data (XRD) resolves ambiguities .
Advanced Research Questions
Q. How can hydrogen-bonding networks in this compound complexes be systematically analyzed?
- Methodological Answer : Single-crystal X-ray diffraction (SC-XRD) is critical. For example, in Co(II)-squarate complexes, squarate dianions form O–H···O and C–H···O bonds with solvent molecules and cations, creating 3D frameworks. Software like WinGX or SIR97 refines hydrogen-bond geometries (bond lengths, angles). Pair distribution function (PDF) analysis supplements SC-XRD for amorphous or polycrystalline samples .
Q. What experimental strategies resolve contradictions in reported decomposition pathways of this compound?
- Methodological Answer : Discrepancies arise from varying hydration states or synthesis conditions. Controlled experiments under inert vs. oxidative atmospheres (using TGA-DSC coupled with EGA) isolate decomposition mechanisms. Replicating studies with identical precursors (e.g., anhydrous Li₂C₄O₄ vs. Li₂C₄O₄·2H₂O) and publishing raw thermal data (e.g., via open-access repositories) enhances reproducibility .
Q. How do computational methods (e.g., DFT) model the electronic structure of this compound?
- Methodological Answer : Density functional theory (DFT) calculations (e.g., using Gaussian or VASP) optimize geometries and compute frontier molecular orbitals. Basis sets like 6-31G* are suitable for squarate’s π-conjugated system. Comparing calculated IR spectra with experimental data validates charge distribution models. For solid-state systems, periodic boundary conditions and hybrid functionals (e.g., PBE0) account for lattice interactions .
Q. What mechanistic insights explain the dichotomous reactivity of squarate derivatives with organolithium reagents?
- Methodological Answer : Kinetic studies (e.g., variable-temperature NMR) track intermediates in reactions like squarate ring-opening. For example, dimethyl squarate reacts with organolithiums via nucleophilic addition (C-attack) or conjugate addition (O-attack), depending on steric/electronic factors. Isotopic labeling (e.g., ¹³C squarate) and DFT-based transition-state analysis clarify pathways .
Q. Data Analysis & Experimental Design
Q. How should researchers design experiments to probe this compound’s role in coordination polymers?
- Methodological Answer : Use a factorial design to vary metal ions (e.g., Li⁺ vs. transition metals), solvent polarity, and counterions. SC-XRD and extended X-ray absorption fine structure (EXAFS) map coordination geometries. Pair with magnetic susceptibility measurements for paramagnetic complexes. Statistical tools (e.g., R or Python’s SciPy) analyze correlations between ligand field strength and polymer dimensionality .
Q. What statistical frameworks are recommended for analyzing spectroscopic data reproducibility?
- Methodological Answer : Principal component analysis (PCA) reduces FTIR/Raman datasets to key variance components. Bootstrapping methods assess confidence intervals for band positions. For conflicting reports, meta-analysis tools (e.g., RevMan) quantify heterogeneity across studies, while Bland-Altman plots evaluate inter-laboratory variability .
Properties
IUPAC Name |
dilithium;3,4-dioxocyclobutene-1,2-diolate | |
---|---|---|
Source | PubChem | |
URL | https://pubchem.ncbi.nlm.nih.gov | |
Description | Data deposited in or computed by PubChem | |
InChI |
InChI=1S/C4H2O4.2Li/c5-1-2(6)4(8)3(1)7;;/h5-6H;;/q;2*+1/p-2 | |
Source | PubChem | |
URL | https://pubchem.ncbi.nlm.nih.gov | |
Description | Data deposited in or computed by PubChem | |
InChI Key |
SOCJEFTZDJUXNO-UHFFFAOYSA-L | |
Source | PubChem | |
URL | https://pubchem.ncbi.nlm.nih.gov | |
Description | Data deposited in or computed by PubChem | |
Canonical SMILES |
[Li+].[Li+].C1(=C(C(=O)C1=O)[O-])[O-] | |
Source | PubChem | |
URL | https://pubchem.ncbi.nlm.nih.gov | |
Description | Data deposited in or computed by PubChem | |
Molecular Formula |
C4Li2O4 | |
Source | PubChem | |
URL | https://pubchem.ncbi.nlm.nih.gov | |
Description | Data deposited in or computed by PubChem | |
Molecular Weight |
126.0 g/mol | |
Source | PubChem | |
URL | https://pubchem.ncbi.nlm.nih.gov | |
Description | Data deposited in or computed by PubChem | |
CAS No. |
104332-28-7 | |
Record name | 3,4-Dihydroxy-3-cyclobutene-1,2-dione dilithium salt | |
Source | European Chemicals Agency (ECHA) | |
URL | https://echa.europa.eu/information-on-chemicals | |
Description | The European Chemicals Agency (ECHA) is an agency of the European Union which is the driving force among regulatory authorities in implementing the EU's groundbreaking chemicals legislation for the benefit of human health and the environment as well as for innovation and competitiveness. | |
Explanation | Use of the information, documents and data from the ECHA website is subject to the terms and conditions of this Legal Notice, and subject to other binding limitations provided for under applicable law, the information, documents and data made available on the ECHA website may be reproduced, distributed and/or used, totally or in part, for non-commercial purposes provided that ECHA is acknowledged as the source: "Source: European Chemicals Agency, http://echa.europa.eu/". Such acknowledgement must be included in each copy of the material. ECHA permits and encourages organisations and individuals to create links to the ECHA website under the following cumulative conditions: Links can only be made to webpages that provide a link to the Legal Notice page. | |
Retrosynthesis Analysis
AI-Powered Synthesis Planning: Our tool employs the Template_relevance Pistachio, Template_relevance Bkms_metabolic, Template_relevance Pistachio_ringbreaker, Template_relevance Reaxys, Template_relevance Reaxys_biocatalysis model, leveraging a vast database of chemical reactions to predict feasible synthetic routes.
One-Step Synthesis Focus: Specifically designed for one-step synthesis, it provides concise and direct routes for your target compounds, streamlining the synthesis process.
Accurate Predictions: Utilizing the extensive PISTACHIO, BKMS_METABOLIC, PISTACHIO_RINGBREAKER, REAXYS, REAXYS_BIOCATALYSIS database, our tool offers high-accuracy predictions, reflecting the latest in chemical research and data.
Strategy Settings
Precursor scoring | Relevance Heuristic |
---|---|
Min. plausibility | 0.01 |
Model | Template_relevance |
Template Set | Pistachio/Bkms_metabolic/Pistachio_ringbreaker/Reaxys/Reaxys_biocatalysis |
Top-N result to add to graph | 6 |
Feasible Synthetic Routes
Disclaimer and Information on In-Vitro Research Products
Please be aware that all articles and product information presented on BenchChem are intended solely for informational purposes. The products available for purchase on BenchChem are specifically designed for in-vitro studies, which are conducted outside of living organisms. In-vitro studies, derived from the Latin term "in glass," involve experiments performed in controlled laboratory settings using cells or tissues. It is important to note that these products are not categorized as medicines or drugs, and they have not received approval from the FDA for the prevention, treatment, or cure of any medical condition, ailment, or disease. We must emphasize that any form of bodily introduction of these products into humans or animals is strictly prohibited by law. It is essential to adhere to these guidelines to ensure compliance with legal and ethical standards in research and experimentation.