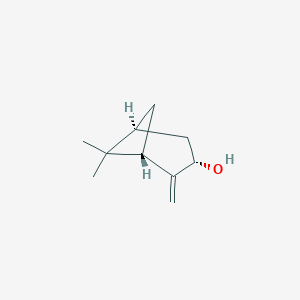
cis-Pinocarveol
Overview
Description
cis-Pinocarveol: is an organic compound with the molecular formula C10H16O . It is a bicyclic monoterpenoid, which means it is derived from two isoprene units and contains a hydroxyl group as a substituent. This compound exists in two stereoisomeric forms: cis and trans, with the cis form being the focus here. This compound is naturally found in various plant species, including Eucalyptus globulus and Picea abies, and is a component of several essential oils .
Preparation Methods
Synthetic Routes and Reaction Conditions: cis-Pinocarveol can be synthesized by heating a mixture of turpentine, selenium dioxide, and hydrogen peroxide. In this reaction, selenium dioxide acts as a catalyst, while hydrogen peroxide oxidizes the pinene found in turpentine. The other components in the turpentine remain unreacted .
Industrial Production Methods: Industrial production of this compound typically involves the same oxidation process using turpentine as the starting material. The reaction conditions are optimized to maximize yield and purity, ensuring that the selenium dioxide catalyst and hydrogen peroxide are used efficiently.
Chemical Reactions Analysis
Types of Reactions: cis-Pinocarveol undergoes various chemical reactions, including:
Oxidation: It can be further oxidized to form pinocarvone.
Reduction: Reduction reactions can convert it back to pinene.
Substitution: The hydroxyl group can be substituted with other functional groups under appropriate conditions.
Common Reagents and Conditions:
Oxidation: Common oxidizing agents include potassium permanganate and chromium trioxide.
Reduction: Reducing agents such as lithium aluminum hydride or sodium borohydride are used.
Substitution: Acidic or basic conditions can facilitate substitution reactions, depending on the desired product.
Major Products:
Oxidation: Pinocarvone
Reduction: Pinene
Substitution: Various substituted derivatives depending on the reagents used
Scientific Research Applications
cis-Pinocarveol has several scientific research applications:
Chemistry: It is used as a chiral building block in the synthesis of complex organic molecules.
Biology: Studies have explored its role in plant defense mechanisms and its potential as a natural insect repellent.
Medicine: Research is ongoing into its potential therapeutic properties, including anti-inflammatory and antimicrobial effects.
Mechanism of Action
The mechanism by which cis-Pinocarveol exerts its effects involves its interaction with various molecular targets and pathways. For instance, its insect-repellent properties are linked to its ability to activate specific receptors in insects, leading to aversion behaviors. In biological systems, it may interact with enzymes and receptors involved in inflammatory and microbial pathways, contributing to its potential therapeutic effects .
Comparison with Similar Compounds
trans-Pinocarveol: The trans isomer of pinocarveol, differing in the stereochemical orientation of the hydroxyl group.
Isopinocarveol: Another stereoisomer with a different arrangement of atoms.
Pinocarvone: An oxidized form of pinocarveol.
Uniqueness: cis-Pinocarveol is unique due to its specific stereochemistry, which can influence its reactivity and interactions with biological systems. Its presence in essential oils and its role in natural plant defense mechanisms also distinguish it from other similar compounds .
Biological Activity
Cis-Pinocarveol is a monoterpenoid alcohol primarily found in various essential oils, particularly those derived from conifers and certain herbs. Its structural formula is , and it is known for its distinctive aroma and potential biological activities. This article delves into the biological activity of this compound, focusing on its antimicrobial, antioxidant, and anti-inflammatory properties, supported by various studies and data.
Chemical Composition
This compound is often present alongside other terpenoids in essential oils. The following table summarizes the chemical composition of some essential oils containing this compound:
Compound | Source | Percentage (%) |
---|---|---|
This compound | Eucalyptus spp. | 4.2 |
Linalool | Eucalyptus spp. | 7.6 |
Camphor | Eucalyptus spp. | 3.5 |
α-Terpinyl Acetate | Eucalyptus spp. | 1.9 |
Sabina ketone | Various | 2.0 |
Antimicrobial Activity
Numerous studies have evaluated the antimicrobial properties of this compound against various pathogens. For instance, a study on essential oils from Siparuna muricata demonstrated significant antimicrobial activity against Staphylococcus aureus, Bacillus cereus, and Candida albicans. The minimum inhibitory concentration (MIC) for this compound was found to be effective at low concentrations, indicating its potential as a natural preservative or therapeutic agent .
Antioxidant Properties
This compound exhibits notable antioxidant activity, which is crucial for combating oxidative stress in biological systems. In a study assessing the antioxidant capacity of various essential oils, this compound contributed to a significant reduction in free radicals, with an IC50 value indicating strong activity . The antioxidant effects were attributed to its ability to scavenge reactive oxygen species (ROS), thereby protecting cells from oxidative damage.
Anti-inflammatory Effects
The anti-inflammatory properties of this compound have been highlighted in several studies. It has been shown to inhibit pro-inflammatory cytokines and enzymes involved in the inflammatory response. A particular study noted that essential oils containing this compound demonstrated higher anti-inflammatory activity during specific seasons, suggesting that environmental factors may influence its efficacy .
Case Studies
- Eucalyptus Essential Oil : A comprehensive analysis of Eucalyptus essential oil revealed that its high content of this compound correlates with its effectiveness against respiratory pathogens, making it a candidate for therapeutic use in respiratory conditions .
- Antimicrobial Testing : In laboratory settings, this compound was tested against various microbial strains using a microtiter plate method, confirming its efficacy with MIC values comparable to conventional antibiotics .
- Seasonal Variation Study : Research indicated that the biological activity of essential oils containing this compound varies seasonally, with peak activities observed during colder months due to higher concentrations of active compounds .
Properties
IUPAC Name |
(1S,3S,5S)-6,6-dimethyl-2-methylidenebicyclo[3.1.1]heptan-3-ol | |
---|---|---|
Source | PubChem | |
URL | https://pubchem.ncbi.nlm.nih.gov | |
Description | Data deposited in or computed by PubChem | |
InChI |
InChI=1S/C10H16O/c1-6-8-4-7(5-9(6)11)10(8,2)3/h7-9,11H,1,4-5H2,2-3H3/t7-,8+,9-/m0/s1 | |
Source | PubChem | |
URL | https://pubchem.ncbi.nlm.nih.gov | |
Description | Data deposited in or computed by PubChem | |
InChI Key |
LCYXQUJDODZYIJ-YIZRAAEISA-N | |
Source | PubChem | |
URL | https://pubchem.ncbi.nlm.nih.gov | |
Description | Data deposited in or computed by PubChem | |
Canonical SMILES |
CC1(C2CC1C(=C)C(C2)O)C | |
Source | PubChem | |
URL | https://pubchem.ncbi.nlm.nih.gov | |
Description | Data deposited in or computed by PubChem | |
Isomeric SMILES |
CC1([C@H]2C[C@@H]1C(=C)[C@H](C2)O)C | |
Source | PubChem | |
URL | https://pubchem.ncbi.nlm.nih.gov | |
Description | Data deposited in or computed by PubChem | |
Molecular Formula |
C10H16O | |
Source | PubChem | |
URL | https://pubchem.ncbi.nlm.nih.gov | |
Description | Data deposited in or computed by PubChem | |
DSSTOX Substance ID |
DTXSID501185680 | |
Record name | (1S,3S,5S)-6,6-Dimethyl-2-methylenebicyclo[3.1.1]heptan-3-ol | |
Source | EPA DSSTox | |
URL | https://comptox.epa.gov/dashboard/DTXSID501185680 | |
Description | DSSTox provides a high quality public chemistry resource for supporting improved predictive toxicology. | |
Molecular Weight |
152.23 g/mol | |
Source | PubChem | |
URL | https://pubchem.ncbi.nlm.nih.gov | |
Description | Data deposited in or computed by PubChem | |
CAS No. |
19889-99-7 | |
Record name | (1S,3S,5S)-6,6-Dimethyl-2-methylenebicyclo[3.1.1]heptan-3-ol | |
Source | CAS Common Chemistry | |
URL | https://commonchemistry.cas.org/detail?cas_rn=19889-99-7 | |
Description | CAS Common Chemistry is an open community resource for accessing chemical information. Nearly 500,000 chemical substances from CAS REGISTRY cover areas of community interest, including common and frequently regulated chemicals, and those relevant to high school and undergraduate chemistry classes. This chemical information, curated by our expert scientists, is provided in alignment with our mission as a division of the American Chemical Society. | |
Explanation | The data from CAS Common Chemistry is provided under a CC-BY-NC 4.0 license, unless otherwise stated. | |
Record name | (1S-(1alpha,3beta,5alpha))-6,6-Dimethyl-2-methylenebicyclo(3.1.1)heptan-3-ol | |
Source | ChemIDplus | |
URL | https://pubchem.ncbi.nlm.nih.gov/substance/?source=chemidplus&sourceid=0019889997 | |
Description | ChemIDplus is a free, web search system that provides access to the structure and nomenclature authority files used for the identification of chemical substances cited in National Library of Medicine (NLM) databases, including the TOXNET system. | |
Record name | (1S,3S,5S)-6,6-Dimethyl-2-methylenebicyclo[3.1.1]heptan-3-ol | |
Source | EPA DSSTox | |
URL | https://comptox.epa.gov/dashboard/DTXSID501185680 | |
Description | DSSTox provides a high quality public chemistry resource for supporting improved predictive toxicology. | |
Record name | [1S-(1α,3β,5α)]-6,6-dimethyl-2-methylenebicyclo[3.1.1]heptan-3-ol | |
Source | European Chemicals Agency (ECHA) | |
URL | https://echa.europa.eu/substance-information/-/substanceinfo/100.039.445 | |
Description | The European Chemicals Agency (ECHA) is an agency of the European Union which is the driving force among regulatory authorities in implementing the EU's groundbreaking chemicals legislation for the benefit of human health and the environment as well as for innovation and competitiveness. | |
Explanation | Use of the information, documents and data from the ECHA website is subject to the terms and conditions of this Legal Notice, and subject to other binding limitations provided for under applicable law, the information, documents and data made available on the ECHA website may be reproduced, distributed and/or used, totally or in part, for non-commercial purposes provided that ECHA is acknowledged as the source: "Source: European Chemicals Agency, http://echa.europa.eu/". Such acknowledgement must be included in each copy of the material. ECHA permits and encourages organisations and individuals to create links to the ECHA website under the following cumulative conditions: Links can only be made to webpages that provide a link to the Legal Notice page. | |
Retrosynthesis Analysis
AI-Powered Synthesis Planning: Our tool employs the Template_relevance Pistachio, Template_relevance Bkms_metabolic, Template_relevance Pistachio_ringbreaker, Template_relevance Reaxys, Template_relevance Reaxys_biocatalysis model, leveraging a vast database of chemical reactions to predict feasible synthetic routes.
One-Step Synthesis Focus: Specifically designed for one-step synthesis, it provides concise and direct routes for your target compounds, streamlining the synthesis process.
Accurate Predictions: Utilizing the extensive PISTACHIO, BKMS_METABOLIC, PISTACHIO_RINGBREAKER, REAXYS, REAXYS_BIOCATALYSIS database, our tool offers high-accuracy predictions, reflecting the latest in chemical research and data.
Strategy Settings
Precursor scoring | Relevance Heuristic |
---|---|
Min. plausibility | 0.01 |
Model | Template_relevance |
Template Set | Pistachio/Bkms_metabolic/Pistachio_ringbreaker/Reaxys/Reaxys_biocatalysis |
Top-N result to add to graph | 6 |
Feasible Synthetic Routes
Q1: What is the primary source of cis-pinocarveol and how is it typically obtained?
A1: this compound is a natural monoterpene alcohol found in the essential oils of various plants. A significant source is Artemisia herba-alba Asso, a plant common to Iranian rangelands. [, ] Steam distillation is a frequently used method for extracting essential oils, with subsequent analysis and identification of this compound often performed using techniques like Gas Chromatography-Mass Spectrometry (GC-MS). [, ]
Q2: Has the chemical composition of essential oils containing this compound been shown to vary?
A2: Yes, research indicates that both the growth stage and altitude of Artemisia herba-alba Asso can influence the essential oil content and composition. For example, higher altitudes (1280m) yielded greater essential oil content, and the relative levels of certain constituents, including this compound, were shown to increase during the flowering stage. [] Similarly, comparative analysis of male and female Hedyosmum scabrum leaves revealed differences in the essential oil composition. Notably, the female leaves exhibited a higher percentage of linalool, while the male leaves contained a greater percentage of pinocarvone. []
Q3: Beyond its presence in essential oils, have any other interesting chemical properties of this compound been investigated?
A3: Yes, this compound has been subjected to pyrolysis, a process of thermal decomposition. This process leads to significant molecular rearrangement, resulting in the formation of an acyclic aldehyde. Deuterium labeling experiments were instrumental in elucidating the mechanism by which this transformation occurs. [, ]
Q4: Are there any known instances of this compound being chemically modified or incorporated into other molecules?
A5: Yes, analysis of Hedyosmum scabrum leaves revealed the presence of non-volatile esters, specifically esters of p-coumaric and ferulic acids with this compound. These findings were made possible through basic hydrolysis of leaf extracts, followed by NMR spectroscopy of the resulting acids and GC-MS analysis of the monoterpene alcohols. [] The identification of these esters expands the understanding of this compound's potential chemical diversity and its role within plant systems.
Disclaimer and Information on In-Vitro Research Products
Please be aware that all articles and product information presented on BenchChem are intended solely for informational purposes. The products available for purchase on BenchChem are specifically designed for in-vitro studies, which are conducted outside of living organisms. In-vitro studies, derived from the Latin term "in glass," involve experiments performed in controlled laboratory settings using cells or tissues. It is important to note that these products are not categorized as medicines or drugs, and they have not received approval from the FDA for the prevention, treatment, or cure of any medical condition, ailment, or disease. We must emphasize that any form of bodily introduction of these products into humans or animals is strictly prohibited by law. It is essential to adhere to these guidelines to ensure compliance with legal and ethical standards in research and experimentation.