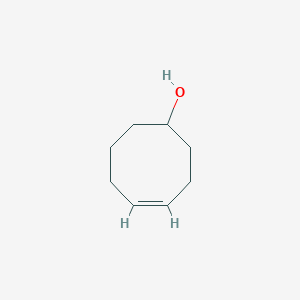
Cyclooct-4-enol
Overview
Description
Cyclooct-4-enol (CAS: 4277-34-3) is an eight-membered cyclic alcohol with a double bond at the 4-position. Its trans-isomer, rel-(1R,4E,pR)-cyclooct-4-enol (TCO-OH; CAS: 85081-69-2), is a strained alkene widely used in bioorthogonal chemistry due to its rapid reactivity with tetrazines via inverse electron-demand Diels-Alder (IEDDA) reactions . The trans configuration introduces significant ring strain, enhancing its kinetic reactivity compared to the cis-isomer (Z-cyclooct-4-enol), which adopts a less strained "crown" conformation .
Preparation Methods
Synthetic Routes and Reaction Conditions: Cyclooct-4-enol can be synthesized through several methods. One common approach involves the reduction of 9-oxabicyclo[6.1.0]non-4-ene using lithium aluminum hydride in diethyl ether. The reaction is typically carried out at room temperature for about four hours, followed by a sequential addition of water and sodium hydroxide to work up the reaction .
Another method involves the photoisomerization of cis-cyclooct-4-enol to its trans isomer using ethyl benzoate as a sensitizer in a two-phase cyclohexane/water system. This process involves irradiation with UV light, which facilitates the isomerization .
Industrial Production Methods: Industrial production of this compound often employs similar synthetic routes but on a larger scale. The use of flow chemistry setups for photoisomerization has been reported to be efficient and cost-effective, allowing for the continuous production of trans-cyclooct-4-enol .
Chemical Reactions Analysis
Types of Reactions: Cyclooct-4-enol undergoes various chemical reactions, including:
Oxidation: It can be oxidized to form cyclooct-4-enone.
Reduction: Reduction reactions can convert it to cyclooctanol.
Substitution: It can participate in substitution reactions, particularly in the presence of strong nucleophiles.
Common Reagents and Conditions:
Oxidation: Common oxidizing agents include potassium permanganate and chromium trioxide.
Reduction: Lithium aluminum hydride and sodium borohydride are frequently used reducing agents.
Substitution: Strong bases like sodium hydride can facilitate substitution reactions.
Major Products:
Oxidation: Cyclooct-4-enone.
Reduction: Cyclooctanol.
Substitution: Various substituted this compound derivatives depending on the nucleophile used.
Scientific Research Applications
Synthetic Chemistry Applications
1.1 Photoisomerization Techniques
One of the notable applications of cyclooct-4-enol is in the photoisomerization process. A recent study proposed a simplified method for converting cis-cyclooct-4-enol to its trans isomer using a two-phase system involving ethyl benzoate as a sensitizer. This approach demonstrated a 3 to 5-fold increase in yield compared to traditional methods, optimizing conditions for maximum photoisomerization efficiency .
Table 1: Comparison of Yields in Photoisomerization Methods
Method | Yield Increase | Notes |
---|---|---|
Traditional Methods | Baseline | Complex setup required |
Proposed Two-Phase Method | 3-5 times | Simple setup, better control over conditions |
1.2 Synthesis of Reactive Dienophiles
This compound derivatives have been utilized to create highly reactive dienophiles for Diels-Alder reactions. Computational studies have shown that trans-cyclooctene derivatives exhibit enhanced reactivity compared to their parent compounds. For instance, a strained bicyclo[6.1.0]non-4-ene derived from this compound was found to be 160 times more reactive than trans-cyclooctene in specific ligation reactions .
Bioorthogonal Chemistry
This compound plays a significant role in bioorthogonal reactions, which are crucial for applications in chemical biology and materials science. Its derivatives are used for efficient labeling and imaging in biological systems due to their compatibility with biological functionalities.
2.1 Applications in Molecular Imaging
Research has indicated that this compound derivatives can improve the pharmacokinetics of imaging agents, facilitating better brain uptake and enhancing their effectiveness in molecular imaging within the central nervous system (CNS) . The use of these compounds allows for precise targeting and visualization of biological processes.
Table 2: Properties of this compound Derivatives in Bioorthogonal Reactions
Compound | Reactivity Enhancement | Application Area |
---|---|---|
Strained Bicyclo[6.1.0]non-4-ene | 160 times | Bioorthogonal ligations |
(E)-Cyclooct-4-enol | High | Biological labeling/imaging |
Materials Science
The unique structural characteristics of this compound make it valuable in materials science, particularly in developing new polymers and nanomaterials.
3.1 Development of Nanoparticles
Recent studies have explored the use of this compound in the synthesis of colloidal gold nanoparticles (AuNPs) that can be functionalized for therapeutic applications. This research demonstrates the potential for this compound derivatives to enhance the efficacy of nanoparticle-based therapies through improved targeting mechanisms .
Mechanism of Action
The primary mechanism of action of cyclooct-4-enol involves its participation in bioorthogonal reactions, particularly the inverse electron demand Diels-Alder cycloaddition with tetrazines. This reaction is highly selective and rapid, making it suitable for in vivo applications. The strained ring structure of this compound increases its reactivity, allowing it to form stable covalent linkages with tetrazine-functionalized compounds .
Comparison with Similar Compounds
Cyclooct-4-enol is compared to other strained alkenes and dienophiles in bioorthogonal chemistry, focusing on reactivity, stability, and synthetic feasibility.
Reactivity with Tetrazines
Compound | Rate Constant (M⁻¹s⁻¹) | Conditions | Reference |
---|---|---|---|
(E)-Cyclooct-4-enol | 92 ± 6 | Methanol, 25°C | |
Methylcyclopropene | 7 ± 1 | 12% DMSO, 37°C | |
Bicyclo[6.1.0]non-4-ene* | Predicted higher | Computational model |
Key Findings:
- TCO-OH exhibits ~13× faster kinetics than methylcyclopropene, making it superior for rapid labeling applications .
- Bicyclo[6.1.0]non-4-ene derivatives, though synthetically challenging, are predicted to exceed TCO-OH’s reactivity due to greater strain .
Stability
Key Findings:
Biological Activity
Cyclooct-4-enol, a cyclic unsaturated alcohol, has garnered attention in recent years due to its unique structural properties and potential applications in various biological contexts. This article explores the biological activity of this compound, focusing on its interactions, mechanisms of action, and implications for therapeutic applications.
This compound is characterized by a cyclooctene ring with a hydroxyl group at the 4-position. Its structural formula can be represented as follows:
The compound exhibits both hydrophilic and hydrophobic characteristics due to the presence of the hydroxyl group and the cyclic structure, which influences its reactivity and biological interactions.
1. Photoactivity and Cytotoxicity
Recent studies have demonstrated that this compound can enhance the cytotoxic effects of certain metal complexes under light irradiation. For instance, when combined with rhenium(I) complexes, this compound significantly increases singlet oxygen generation, leading to enhanced photodynamic therapy (PDT) efficacy against cancer cells. In a study involving MDA-MB-231 breast cancer cells, treatment with a rhenium complex in the presence of this compound resulted in increased necrosis and reduced cell viability upon light exposure .
Table 1: Cytotoxicity Data of Rhenium Complexes with this compound
Treatment Condition | IC50 (μM) Dark | IC50 (μM) Light |
---|---|---|
Rhenium Complex Alone | 51.1–73.7 | 15.5–20.9 |
Rhenium Complex + TCO-OH | 3.4–35.6 | 18.3–1.4 |
This data indicates that this compound enhances the cytotoxicity of rhenium complexes when exposed to light, suggesting potential applications in targeted cancer therapies.
2. Reactivity in Bioorthogonal Chemistry
This compound is also recognized for its utility in bioorthogonal reactions, particularly involving strained alkene chemistry. Its ability to participate in inverse electron-demand Diels-Alder (IEDDA) reactions makes it a valuable tool for bioconjugation strategies . The strain within the cyclooctene structure facilitates rapid reactions with dienophiles, making it an effective candidate for labeling biomolecules in live cells.
Table 2: Reactivity Comparison of Cycloalkenes
Compound | Reactivity (Relative Rate) |
---|---|
Cyclopropene | Highest |
Cyclobutene | High |
Cyclopentene | Moderate |
Cyclohexene | Lower |
Cyclooctene | Increased |
The mechanisms underlying the biological activity of this compound involve several pathways:
- Singlet Oxygen Generation : The interaction of this compound with photosensitizers leads to enhanced production of singlet oxygen, which is cytotoxic to cells.
- Cell Death Pathways : Studies indicate that treatment with this compound in conjunction with light exposure primarily induces necrosis in cancer cells, as evidenced by increased Annexin V/PI staining .
Case Study 1: Photodynamic Therapy Enhancement
In a controlled experiment, MDA-MB-231 cells were treated with a rhenium complex and this compound under light irradiation. The results showed a significant increase in necrotic cell populations compared to controls without this compound, highlighting its role as an effective sensitizer in PDT .
Case Study 2: Bioorthogonal Labeling
In another study focusing on bioorthogonal chemistry, this compound was utilized to label antibodies in vivo. The high reactivity of cycloalkenes allowed for efficient conjugation without compromising biological activity, demonstrating its potential in therapeutic applications .
Q & A
Q. What are the key experimental considerations for synthesizing (Z)-cyclooct-4-enol, and how can reproducibility be ensured?
Basic Research Question
The synthesis of (Z)-cyclooct-4-enol typically involves lithium aluminium hydride (LiAlH₄) reduction of 1,2-epoxy-5-cyclooctene in dry tetrahydrofuran (THF) under argon, yielding 99% purity . To ensure reproducibility:
- Use oven-dried glassware and rigorously exclude moisture.
- Monitor reaction progress via <sup>1</sup>H and <sup>13</sup>C NMR to confirm product identity and purity, comparing spectral data to literature values .
- Document quenching and extraction protocols (e.g., Rochelle salt for safe LiAlH₄ neutralization) .
Q. How can researchers resolve contradictions in isomerization efficiency between (Z)- and (E)-cyclooct-4-enol synthesis methods?
Advanced Research Question
Contradictions often arise from sensitizer choice, solvent systems, or irradiation conditions. For example, ethyl benzoate sensitization in a cyclohexane/water biphasic system improves (E)-isomer yield via continuous silver complex extraction . Methodological steps to address discrepancies:
- Compare thermodynamic parameters (e.g., distribution constant KD = 0.92 ± 0.05 for cyclooct-4-enol in cyclohexane/water) .
- Optimize photolysis conditions using UV-Vis spectroscopy to monitor stability constants (KAgZ = 2.5 M⁻¹ vs. KAgE = 2100 M⁻¹) .
- Validate with independent techniques like inverse electron demand Diels–Alder (IEDDA) reactivity assays .
Q. What PICOT framework elements are critical for designing studies on this compound’s bioorthogonal applications?
Methodological Guidance
Using the PICOT framework (Population, Intervention, Comparison, Outcome, Time):
- Population : Specific biomolecules or cellular targets (e.g., tetrazine-functionalized proteins).
- Intervention : Bioorthogonal click reactions using (E)-cyclooct-4-enol due to its higher IEDDA reactivity .
- Comparison : Contrast with other dienophiles (e.g., cis-cyclooctene) to highlight kinetic advantages.
- Outcome : Quantify labeling efficiency via fluorescence or mass spectrometry.
- Time : Reaction kinetics under physiological conditions (e.g., 37°C, pH 7.4) .
This ensures alignment with reproducibility and clinical relevance criteria .
Q. How can researchers mitigate safety risks when handling this compound in laboratory settings?
Basic Research Question
- Use PPE (gloves, goggles, lab coats) and work in a fume hood to avoid dermal/ocular exposure .
- Store waste separately in labeled containers for professional disposal due to potential environmental toxicity .
- Prefer non-hazardous solvents (e.g., cyclohexane over halogenated solvents) in photoisomerization protocols .
Q. What statistical and analytical methods are recommended for quantifying this compound isomer ratios in complex mixtures?
Advanced Research Question
- Chromatography : HPLC or GC-MS with chiral columns to separate (Z)- and (E)-isomers .
- Spectroscopy : UV-Vis analysis (200 nm absorbance) to determine distribution constants in biphasic systems .
- Error Analysis : Report standard deviations for stability constants (e.g., KAgE = 2100 ± 100 M⁻¹) and use t-tests to compare experimental vs. literature values .
Q. How can thermodynamic modeling improve the scalability of this compound photoisomerization?
Advanced Research Question
- Develop models incorporating stability constants (KAgZ, KAgE), distribution coefficients (KD), and irradiation intensity to predict yield .
- Validate with experimental titration data (e.g., AgNO3 concentration vs. isomer extraction efficiency) .
- Optimize solvent ratios (cyclohexane/water) to maximize continuous extraction of the silver-complexed (E)-isomer .
Q. What ethical considerations apply to in vivo studies using this compound-based probes?
Methodological Guidance
- Animal Studies : Minimize sample size using power analysis and adhere to the 3Rs (Replacement, Reduction, Refinement) .
- Human Trials : Obtain IRB approval, disclose risks in informed consent, and ensure biocompatibility (e.g., low cytotoxicity at <1 mM concentrations) .
Q. How do structural modifications of this compound impact its reactivity in bioorthogonal chemistry?
Advanced Research Question
- Steric Effects : Introduce substituents (e.g., methyl groups) to assess strain-enhanced reactivity in IEDDA reactions .
- Electronic Effects : Compare electron-withdrawing vs. donating groups using Hammett plots to correlate reactivity with substituent constants.
- Validate with kinetic assays (e.g., second-order rate constants k2 measured via stopped-flow spectroscopy) .
Properties
IUPAC Name |
(4Z)-cyclooct-4-en-1-ol | |
---|---|---|
Source | PubChem | |
URL | https://pubchem.ncbi.nlm.nih.gov | |
Description | Data deposited in or computed by PubChem | |
InChI |
InChI=1S/C8H14O/c9-8-6-4-2-1-3-5-7-8/h1-2,8-9H,3-7H2/b2-1- | |
Source | PubChem | |
URL | https://pubchem.ncbi.nlm.nih.gov | |
Description | Data deposited in or computed by PubChem | |
InChI Key |
UCPDHOTYYDHPEN-UPHRSURJSA-N | |
Source | PubChem | |
URL | https://pubchem.ncbi.nlm.nih.gov | |
Description | Data deposited in or computed by PubChem | |
Canonical SMILES |
C1CC=CCCC(C1)O | |
Source | PubChem | |
URL | https://pubchem.ncbi.nlm.nih.gov | |
Description | Data deposited in or computed by PubChem | |
Isomeric SMILES |
C1C/C=C\CCC(C1)O | |
Source | PubChem | |
URL | https://pubchem.ncbi.nlm.nih.gov | |
Description | Data deposited in or computed by PubChem | |
Molecular Formula |
C8H14O | |
Source | PubChem | |
URL | https://pubchem.ncbi.nlm.nih.gov | |
Description | Data deposited in or computed by PubChem | |
DSSTOX Substance ID |
DTXSID701316411 | |
Record name | 4-Cycloocten-1-ol | |
Source | EPA DSSTox | |
URL | https://comptox.epa.gov/dashboard/DTXSID701316411 | |
Description | DSSTox provides a high quality public chemistry resource for supporting improved predictive toxicology. | |
Molecular Weight |
126.20 g/mol | |
Source | PubChem | |
URL | https://pubchem.ncbi.nlm.nih.gov | |
Description | Data deposited in or computed by PubChem | |
CAS No. |
4277-34-3, 85081-69-2 | |
Record name | 4-Cycloocten-1-ol | |
Source | CAS Common Chemistry | |
URL | https://commonchemistry.cas.org/detail?cas_rn=4277-34-3 | |
Description | CAS Common Chemistry is an open community resource for accessing chemical information. Nearly 500,000 chemical substances from CAS REGISTRY cover areas of community interest, including common and frequently regulated chemicals, and those relevant to high school and undergraduate chemistry classes. This chemical information, curated by our expert scientists, is provided in alignment with our mission as a division of the American Chemical Society. | |
Explanation | The data from CAS Common Chemistry is provided under a CC-BY-NC 4.0 license, unless otherwise stated. | |
Record name | Cyclooct-4-en-1-ol | |
Source | ChemIDplus | |
URL | https://pubchem.ncbi.nlm.nih.gov/substance/?source=chemidplus&sourceid=0004277343 | |
Description | ChemIDplus is a free, web search system that provides access to the structure and nomenclature authority files used for the identification of chemical substances cited in National Library of Medicine (NLM) databases, including the TOXNET system. | |
Record name | 4277-34-3 | |
Source | DTP/NCI | |
URL | https://dtp.cancer.gov/dtpstandard/servlet/dwindex?searchtype=NSC&outputformat=html&searchlist=119487 | |
Description | The NCI Development Therapeutics Program (DTP) provides services and resources to the academic and private-sector research communities worldwide to facilitate the discovery and development of new cancer therapeutic agents. | |
Explanation | Unless otherwise indicated, all text within NCI products is free of copyright and may be reused without our permission. Credit the National Cancer Institute as the source. | |
Record name | 4-Cycloocten-1-ol | |
Source | EPA DSSTox | |
URL | https://comptox.epa.gov/dashboard/DTXSID701316411 | |
Description | DSSTox provides a high quality public chemistry resource for supporting improved predictive toxicology. | |
Record name | Cyclooct-4-en-1-ol | |
Source | European Chemicals Agency (ECHA) | |
URL | https://echa.europa.eu/substance-information/-/substanceinfo/100.022.073 | |
Description | The European Chemicals Agency (ECHA) is an agency of the European Union which is the driving force among regulatory authorities in implementing the EU's groundbreaking chemicals legislation for the benefit of human health and the environment as well as for innovation and competitiveness. | |
Explanation | Use of the information, documents and data from the ECHA website is subject to the terms and conditions of this Legal Notice, and subject to other binding limitations provided for under applicable law, the information, documents and data made available on the ECHA website may be reproduced, distributed and/or used, totally or in part, for non-commercial purposes provided that ECHA is acknowledged as the source: "Source: European Chemicals Agency, http://echa.europa.eu/". Such acknowledgement must be included in each copy of the material. ECHA permits and encourages organisations and individuals to create links to the ECHA website under the following cumulative conditions: Links can only be made to webpages that provide a link to the Legal Notice page. | |
Record name | 85081-69-2 | |
Source | European Chemicals Agency (ECHA) | |
URL | https://echa.europa.eu/information-on-chemicals | |
Description | The European Chemicals Agency (ECHA) is an agency of the European Union which is the driving force among regulatory authorities in implementing the EU's groundbreaking chemicals legislation for the benefit of human health and the environment as well as for innovation and competitiveness. | |
Explanation | Use of the information, documents and data from the ECHA website is subject to the terms and conditions of this Legal Notice, and subject to other binding limitations provided for under applicable law, the information, documents and data made available on the ECHA website may be reproduced, distributed and/or used, totally or in part, for non-commercial purposes provided that ECHA is acknowledged as the source: "Source: European Chemicals Agency, http://echa.europa.eu/". Such acknowledgement must be included in each copy of the material. ECHA permits and encourages organisations and individuals to create links to the ECHA website under the following cumulative conditions: Links can only be made to webpages that provide a link to the Legal Notice page. | |
Retrosynthesis Analysis
AI-Powered Synthesis Planning: Our tool employs the Template_relevance Pistachio, Template_relevance Bkms_metabolic, Template_relevance Pistachio_ringbreaker, Template_relevance Reaxys, Template_relevance Reaxys_biocatalysis model, leveraging a vast database of chemical reactions to predict feasible synthetic routes.
One-Step Synthesis Focus: Specifically designed for one-step synthesis, it provides concise and direct routes for your target compounds, streamlining the synthesis process.
Accurate Predictions: Utilizing the extensive PISTACHIO, BKMS_METABOLIC, PISTACHIO_RINGBREAKER, REAXYS, REAXYS_BIOCATALYSIS database, our tool offers high-accuracy predictions, reflecting the latest in chemical research and data.
Strategy Settings
Precursor scoring | Relevance Heuristic |
---|---|
Min. plausibility | 0.01 |
Model | Template_relevance |
Template Set | Pistachio/Bkms_metabolic/Pistachio_ringbreaker/Reaxys/Reaxys_biocatalysis |
Top-N result to add to graph | 6 |
Feasible Synthetic Routes
Disclaimer and Information on In-Vitro Research Products
Please be aware that all articles and product information presented on BenchChem are intended solely for informational purposes. The products available for purchase on BenchChem are specifically designed for in-vitro studies, which are conducted outside of living organisms. In-vitro studies, derived from the Latin term "in glass," involve experiments performed in controlled laboratory settings using cells or tissues. It is important to note that these products are not categorized as medicines or drugs, and they have not received approval from the FDA for the prevention, treatment, or cure of any medical condition, ailment, or disease. We must emphasize that any form of bodily introduction of these products into humans or animals is strictly prohibited by law. It is essential to adhere to these guidelines to ensure compliance with legal and ethical standards in research and experimentation.