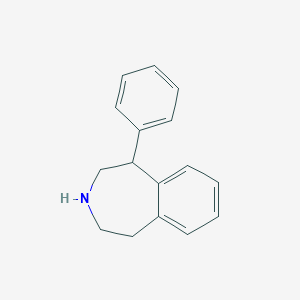
1-phenyl-2,3,4,5-tetrahydro-1H-3-benzazepine
Overview
Description
1-phenyl-2,3,4,5-tetrahydro-1H-3-benzazepine is a useful research compound. Its molecular formula is C16H17N and its molecular weight is 223.31 g/mol. The purity is usually 95%.
BenchChem offers high-quality this compound suitable for many research applications. Different packaging options are available to accommodate customers' requirements. Please inquire for more information about this compound including the price, delivery time, and more detailed information at info@benchchem.com.
Mechanism of Action
Target of Action
1-Phenyl-2,3,4,5-tetrahydro-1H-3-benzazepine is a benzazepine derivative Related compounds have been found to interact with d1 dopamine receptors .
Mode of Action
Structurally related compounds have been found to block g protein-coupled inwardly rectifying potassium (girk) channels . The halide atom in these compounds is critical for this blocking action .
Biochemical Pathways
The interaction with d1 dopamine receptors and girk channels suggests that it may influence neurotransmission .
Result of Action
The blocking of girk channels suggests that it may influence cellular excitability .
Biochemical Analysis
Biochemical Properties
1-phenyl-2,3,4,5-tetrahydro-1H-3-benzazepine plays a significant role in biochemical reactions, particularly in the modulation of neurotransmitter systems. It interacts with various enzymes, proteins, and other biomolecules. One of the primary interactions is with dopamine receptors, where it acts as an agonist or antagonist depending on the specific receptor subtype. This compound has been shown to interact with D1-like dopamine receptors, enhancing excitatory postsynaptic currents (EPSCs) in certain neuronal populations . Additionally, it can inhibit G protein-coupled inwardly rectifying potassium (GIRK) channels, which are involved in regulating neuronal excitability .
Cellular Effects
The effects of this compound on various cell types and cellular processes are profound. In neuronal cells, it influences cell signaling pathways, gene expression, and cellular metabolism. For instance, the compound has been shown to increase the amplitude of EPSCs in pyramidal neurons, indicating its role in modulating synaptic transmission . Furthermore, it can affect the expression of immediate-early genes such as c-fos, which are involved in neuronal plasticity and response to stimuli . These effects highlight the compound’s potential impact on cognitive and behavioral functions.
Molecular Mechanism
At the molecular level, this compound exerts its effects through several mechanisms. It binds to dopamine receptors, particularly the D1-like receptors, and modulates their activity. This binding leads to the activation or inhibition of downstream signaling pathways, including those involving cyclic AMP (cAMP) and protein kinase A (PKA). Additionally, the compound’s ability to inhibit GIRK channels suggests a role in modulating ion channel activity and neuronal excitability . These molecular interactions contribute to the compound’s overall pharmacological profile.
Temporal Effects in Laboratory Settings
In laboratory settings, the effects of this compound can change over time. The compound’s stability and degradation are critical factors that influence its long-term effects on cellular function. Studies have shown that the compound can maintain its activity over extended periods, but its efficacy may diminish due to metabolic degradation . Long-term exposure to the compound in vitro and in vivo has been associated with sustained changes in gene expression and cellular metabolism, indicating potential long-term therapeutic or adverse effects.
Dosage Effects in Animal Models
The effects of this compound vary with different dosages in animal models. At lower doses, the compound may exhibit therapeutic effects, such as enhancing cognitive function and reducing symptoms of neurological disorders. At higher doses, it can lead to toxic or adverse effects, including neurotoxicity and behavioral changes . Threshold effects have been observed, where the compound’s efficacy plateaus or diminishes beyond a certain dosage, highlighting the importance of dose optimization in therapeutic applications.
Metabolic Pathways
This compound is involved in several metabolic pathways. It is metabolized primarily in the liver by enzymes such as cytochrome P450 oxidases. The compound’s metabolites can have varying degrees of activity and may contribute to its overall pharmacological effects . Additionally, the compound can influence metabolic flux and metabolite levels in target tissues, further modulating its biological activity.
Transport and Distribution
The transport and distribution of this compound within cells and tissues are mediated by specific transporters and binding proteins. The compound can cross the blood-brain barrier, allowing it to exert its effects on the central nervous system. Within cells, it may be transported by organic cation transporters and localized to specific subcellular compartments . The distribution of the compound within tissues can influence its efficacy and potential side effects.
Subcellular Localization
The subcellular localization of this compound is crucial for its activity and function. The compound can be directed to specific compartments or organelles through targeting signals or post-translational modifications. For example, it may localize to the plasma membrane, where it interacts with membrane-bound receptors and ion channels . Additionally, its presence in the cytoplasm and nucleus can influence intracellular signaling pathways and gene expression.
Properties
IUPAC Name |
5-phenyl-2,3,4,5-tetrahydro-1H-3-benzazepine | |
---|---|---|
Source | PubChem | |
URL | https://pubchem.ncbi.nlm.nih.gov | |
Description | Data deposited in or computed by PubChem | |
InChI |
InChI=1S/C16H17N/c1-2-6-13(7-3-1)16-12-17-11-10-14-8-4-5-9-15(14)16/h1-9,16-17H,10-12H2 | |
Source | PubChem | |
URL | https://pubchem.ncbi.nlm.nih.gov | |
Description | Data deposited in or computed by PubChem | |
InChI Key |
MEXPXLPUVFBQGJ-UHFFFAOYSA-N | |
Source | PubChem | |
URL | https://pubchem.ncbi.nlm.nih.gov | |
Description | Data deposited in or computed by PubChem | |
Canonical SMILES |
C1CNCC(C2=CC=CC=C21)C3=CC=CC=C3 | |
Source | PubChem | |
URL | https://pubchem.ncbi.nlm.nih.gov | |
Description | Data deposited in or computed by PubChem | |
Molecular Formula |
C16H17N | |
Source | PubChem | |
URL | https://pubchem.ncbi.nlm.nih.gov | |
Description | Data deposited in or computed by PubChem | |
Molecular Weight |
223.31 g/mol | |
Source | PubChem | |
URL | https://pubchem.ncbi.nlm.nih.gov | |
Description | Data deposited in or computed by PubChem | |
Retrosynthesis Analysis
AI-Powered Synthesis Planning: Our tool employs the Template_relevance Pistachio, Template_relevance Bkms_metabolic, Template_relevance Pistachio_ringbreaker, Template_relevance Reaxys, Template_relevance Reaxys_biocatalysis model, leveraging a vast database of chemical reactions to predict feasible synthetic routes.
One-Step Synthesis Focus: Specifically designed for one-step synthesis, it provides concise and direct routes for your target compounds, streamlining the synthesis process.
Accurate Predictions: Utilizing the extensive PISTACHIO, BKMS_METABOLIC, PISTACHIO_RINGBREAKER, REAXYS, REAXYS_BIOCATALYSIS database, our tool offers high-accuracy predictions, reflecting the latest in chemical research and data.
Strategy Settings
Precursor scoring | Relevance Heuristic |
---|---|
Min. plausibility | 0.01 |
Model | Template_relevance |
Template Set | Pistachio/Bkms_metabolic/Pistachio_ringbreaker/Reaxys/Reaxys_biocatalysis |
Top-N result to add to graph | 6 |
Feasible Synthetic Routes
Q1: What is the primary target of 1-phenyl-2,3,4,5-tetrahydro-1H-3-benzazepine and its derivatives?
A1: this compound derivatives primarily target dopamine receptors, specifically the D1 and D2 subtypes, which are crucial for modulating various neurological functions. [, , , ]
Q2: How do different substituents on the this compound scaffold influence its affinity for D1 and D2 receptors?
A2: Substitutions at various positions on the benzazepine ring system significantly affect the compound's affinity and selectivity for D1 and D2 receptors. For example, the presence of a 7-chloro substituent in SCH23390 enhances its antagonist activity at D1 receptors. [, , ] In contrast, 6-chloro derivatives like SKF 82958 display potent D1 agonist activity. [, ]
Q3: What are the downstream effects of activating D1 receptors with agonists like SKF 82958?
A3: Activation of D1 receptors with agonists like SKF 82958 can lead to various downstream effects, including adenylate cyclase activation, increased cAMP production, and modulation of protein kinase A (PKA) activity. [, , , , ] These signaling cascades ultimately influence neuronal excitability, synaptic plasticity, and behavior.
Q4: How does the activation of D2 receptors by this compound derivatives differ from D1 receptor activation?
A4: Unlike D1 agonists, which primarily activate adenylate cyclase, D2 receptor activation typically leads to the inhibition of adenylate cyclase and a decrease in cAMP levels. [, , ] D2 receptor activation can also modulate ion channels like G protein-gated inwardly rectifying potassium (GIRK) channels, influencing neuronal excitability. [, ]
Q5: Can this compound derivatives differentiate between D1 and D2 receptor subtypes?
A5: Yes, specific substitutions on the this compound scaffold impart selectivity for either D1 or D2 receptors. For example, SCH23390 demonstrates high selectivity for D1 receptors, whereas quinpirole displays selectivity for D2 receptors. [, , , , ] This selectivity is crucial for dissecting the individual roles of D1 and D2 receptors in various physiological and behavioral processes.
Q6: What is the significance of co-activation of both D1 and D2 receptors by some this compound derivatives?
A6: Studies have shown that co-activation of D1 and D2 receptors can lead to unique signaling events, such as the activation of phospholipase C (PLC) and subsequent calcium release. [, ] This suggests complex interactions between these receptor subtypes within the dopaminergic system, which might have significant implications for therapeutic interventions.
Q7: How does the 3-position substituent on the this compound scaffold affect its pharmacological activity?
A7: The 3-position substituent plays a significant role in determining the compound's pharmacological properties. For instance, 3-methyl substituted compounds often exhibit greater affinity for dopamine receptors compared to their 3-unsubstituted counterparts. [, ] The presence of a 3-allyl group, as seen in SKF 82958, can further enhance D1 receptor agonist activity and potency. [, ]
Q8: Does the presence of a halogen at the 6- or 7-position of the benzazepine ring system impact the compound's activity?
A8: Yes, halogen substituents at the 6- and 7-positions are crucial for modulating the compound's pharmacological profile. For example, 6-chloro derivatives like SKF 81297 and SKF 82958 show potent D1 agonist activity, whereas the 7-chloro analogue SCH23390 acts as a potent D1 antagonist. [, , ] These findings highlight the importance of halogen substitution in fine-tuning the activity and selectivity of this compound derivatives.
Q9: How does the lipophilicity of this compound derivatives correlate with their ability to cross the blood-brain barrier and exert central nervous system (CNS) effects?
A9: Increased lipophilicity generally enhances the ability of these compounds to penetrate the blood-brain barrier and exert effects within the CNS. [, ] The 3-allyl derivatives, due to their greater lipophilicity compared to 3-methyl analogues, demonstrate enhanced CNS penetration and potentially higher in vivo potency.
Q10: What are some commonly used in vitro models to assess the activity of this compound derivatives?
A10: In vitro models, such as cell lines expressing recombinant dopamine receptors or isolated tissue preparations, are valuable tools for studying the activity of these compounds. For example, receptor binding assays using radiolabeled ligands like [3H]SCH23390 can determine the affinity of test compounds for D1 receptors. [, , ] Additionally, functional assays measuring cAMP accumulation or second messenger production can assess the agonist or antagonist properties of these compounds. [, , ]
Q11: Which animal models have been employed to investigate the behavioral effects of this compound derivatives?
A11: Rodent models, particularly rats and mice, have been extensively used to study the behavioral effects of these compounds. Behavioral paradigms like prepulse inhibition (PPI) of the acoustic startle response and locomotor activity tests are employed to assess the psychomotor stimulant-like effects of D1 and D2 agonists. [, , ] These models have revealed significant species differences in the dopaminergic modulation of behavior, highlighting the importance of choosing appropriate animal models for translational research.
Q12: What insights have been gained from in vivo studies using these compounds to understand the role of dopamine in reward and addiction?
A12: Animal studies using this compound derivatives have been instrumental in dissecting the role of dopamine in reward and addiction. For example, studies using intracranial self-administration paradigms have shown that co-activation of D1 and D2 receptors within the nucleus accumbens shell is reinforcing, highlighting the importance of both receptor subtypes in reward processing. [] Furthermore, research using conditioned place preference (CPP) paradigms has demonstrated that D1 receptor activation contributes to the rewarding effects of drugs like cocaine. [, ]
Disclaimer and Information on In-Vitro Research Products
Please be aware that all articles and product information presented on BenchChem are intended solely for informational purposes. The products available for purchase on BenchChem are specifically designed for in-vitro studies, which are conducted outside of living organisms. In-vitro studies, derived from the Latin term "in glass," involve experiments performed in controlled laboratory settings using cells or tissues. It is important to note that these products are not categorized as medicines or drugs, and they have not received approval from the FDA for the prevention, treatment, or cure of any medical condition, ailment, or disease. We must emphasize that any form of bodily introduction of these products into humans or animals is strictly prohibited by law. It is essential to adhere to these guidelines to ensure compliance with legal and ethical standards in research and experimentation.