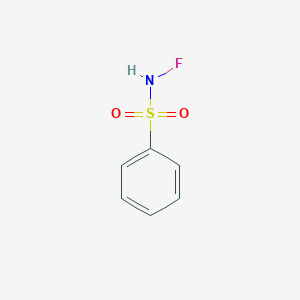
n-Fluorobenzenesulfonamide
Overview
Description
n-Fluorobenzenesulfonamide is a versatile and widely used reagent in organic synthesis. It is particularly known for its role as an electrophilic fluorinating agent. This compound is a stable, crystalline solid that is highly soluble and easy to handle. Its unique properties make it an essential tool in various chemical transformations, especially in the fields of medicinal chemistry, materials science, and agrochemicals.
Scientific Research Applications
n-Fluorobenzenesulfonamide has a wide range of applications in scientific research:
Mechanism of Action
N-Fluorobenzenesulfonimide fluorinates aromatics, enolates, azaenolates, and carbanions in high yield. It is used for diastereoselective fluorination of Li enolates of chiral carboximides, for directed fluorination of ortho-lithiated aromatics, and for a review of electrophilic N-F fluorinating agents .
Safety and Hazards
Future Directions
Recent advances and developments in the direct fluorination and amination of (hetero)aromatic C–H bonds utilizing N-Fluorobenzenesulfonimide have been reported . These developments suggest that N-Fluorobenzenesulfonimide will continue to be a useful and versatile reagent in the field of organic synthesis.
Preparation Methods
Synthetic Routes and Reaction Conditions: n-Fluorobenzenesulfonamide can be synthesized through the reaction of benzenesulfonimide with fluorine gas. The process involves the oxidative addition of palladium (0) to the nitrogen-fluorine bond of this compound, forming an intermediate complex. This intermediate then undergoes further transformations to yield the final product .
Industrial Production Methods: In industrial settings, the production of this compound typically involves the use of safer and more stable fluorinating agents compared to molecular fluorine. These agents include N-fluoropyridinium salts, which offer better handling and selectivity .
Chemical Reactions Analysis
Types of Reactions: n-Fluorobenzenesulfonamide undergoes various types of chemical reactions, including:
Fluorination: It acts as an electrophilic fluorinating agent, introducing fluorine atoms into aromatic and aliphatic compounds.
Common Reagents and Conditions:
Fluorination: Common reagents include palladium catalysts and bases.
Amination: Reagents such as palladium complexes and bases are used under similar mild conditions.
Major Products:
Fluorinated Aromatics: These are the primary products of fluorination reactions.
Aminated Compounds: These result from amination reactions and are valuable intermediates in pharmaceutical synthesis.
Comparison with Similar Compounds
n-Fluorobenzenesulfonamide is unique among fluorinating agents due to its stability, ease of handling, and selectivity. Similar compounds include:
N-Fluoropyridinium Salts: These are also used as electrophilic fluorinating agents but may have different selectivity and handling properties.
N-Fluorobenzenesulfonimide: This compound is closely related and shares similar applications but may differ in reactivity and stability.
Properties
IUPAC Name |
N-fluorobenzenesulfonamide | |
---|---|---|
Source | PubChem | |
URL | https://pubchem.ncbi.nlm.nih.gov | |
Description | Data deposited in or computed by PubChem | |
InChI |
InChI=1S/C6H6FNO2S/c7-8-11(9,10)6-4-2-1-3-5-6/h1-5,8H | |
Source | PubChem | |
URL | https://pubchem.ncbi.nlm.nih.gov | |
Description | Data deposited in or computed by PubChem | |
InChI Key |
CJYQZTZSYREQBD-UHFFFAOYSA-N | |
Source | PubChem | |
URL | https://pubchem.ncbi.nlm.nih.gov | |
Description | Data deposited in or computed by PubChem | |
Canonical SMILES |
C1=CC=C(C=C1)S(=O)(=O)NF | |
Source | PubChem | |
URL | https://pubchem.ncbi.nlm.nih.gov | |
Description | Data deposited in or computed by PubChem | |
Molecular Formula |
C6H6FNO2S | |
Source | PubChem | |
URL | https://pubchem.ncbi.nlm.nih.gov | |
Description | Data deposited in or computed by PubChem | |
DSSTOX Substance ID |
DTXSID80572114 | |
Record name | N-Fluorobenzenesulfonamide | |
Source | EPA DSSTox | |
URL | https://comptox.epa.gov/dashboard/DTXSID80572114 | |
Description | DSSTox provides a high quality public chemistry resource for supporting improved predictive toxicology. | |
Molecular Weight |
175.18 g/mol | |
Source | PubChem | |
URL | https://pubchem.ncbi.nlm.nih.gov | |
Description | Data deposited in or computed by PubChem | |
CAS No. |
145490-75-1 | |
Record name | N-Fluorobenzenesulfonamide | |
Source | EPA DSSTox | |
URL | https://comptox.epa.gov/dashboard/DTXSID80572114 | |
Description | DSSTox provides a high quality public chemistry resource for supporting improved predictive toxicology. | |
Retrosynthesis Analysis
AI-Powered Synthesis Planning: Our tool employs the Template_relevance Pistachio, Template_relevance Bkms_metabolic, Template_relevance Pistachio_ringbreaker, Template_relevance Reaxys, Template_relevance Reaxys_biocatalysis model, leveraging a vast database of chemical reactions to predict feasible synthetic routes.
One-Step Synthesis Focus: Specifically designed for one-step synthesis, it provides concise and direct routes for your target compounds, streamlining the synthesis process.
Accurate Predictions: Utilizing the extensive PISTACHIO, BKMS_METABOLIC, PISTACHIO_RINGBREAKER, REAXYS, REAXYS_BIOCATALYSIS database, our tool offers high-accuracy predictions, reflecting the latest in chemical research and data.
Strategy Settings
Precursor scoring | Relevance Heuristic |
---|---|
Min. plausibility | 0.01 |
Model | Template_relevance |
Template Set | Pistachio/Bkms_metabolic/Pistachio_ringbreaker/Reaxys/Reaxys_biocatalysis |
Top-N result to add to graph | 6 |
Feasible Synthetic Routes
Disclaimer and Information on In-Vitro Research Products
Please be aware that all articles and product information presented on BenchChem are intended solely for informational purposes. The products available for purchase on BenchChem are specifically designed for in-vitro studies, which are conducted outside of living organisms. In-vitro studies, derived from the Latin term "in glass," involve experiments performed in controlled laboratory settings using cells or tissues. It is important to note that these products are not categorized as medicines or drugs, and they have not received approval from the FDA for the prevention, treatment, or cure of any medical condition, ailment, or disease. We must emphasize that any form of bodily introduction of these products into humans or animals is strictly prohibited by law. It is essential to adhere to these guidelines to ensure compliance with legal and ethical standards in research and experimentation.