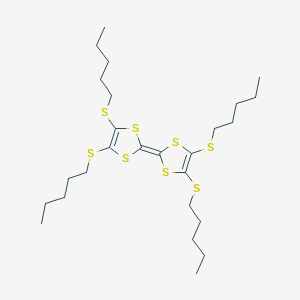
Tpt-ttf
Overview
Description
Tetrathiafulvalene derivative (Tpt-ttf), registered under CAS number 106920-29-0, is a sulfur-rich organic compound with significant applications across multiple industries. Its molecular structure, based on a tetrathiafulvalene (TTF) core with specific substituents, enables unique electronic properties, particularly its ability to form metal charge transfer salts with high conductivity . Key applications include:
- Electronics: Utilized in conductive materials for devices due to its charge-transfer capabilities.
- Chemical Industry: Acts as a precursor for synthesizing organic/metal nanoparticles and drug delivery systems.
- Pharmaceuticals: Enhances chemotherapeutic drug efficacy in anticancer therapies by improving bioavailability and chemical sensitivity .
Preparation Methods
Synthetic Routes and Reaction Conditions
The synthesis of Tetrathiafulvalene-tetrabenzaldehyde typically involves the coupling of cyclic building blocks such as 1,3-dithiole-2-thione or related compounds. The process begins with the methylation of cyclic trithiocarbonate, followed by reduction to form the intermediate 1,3-dithiole-2-yl methyl thioether. This intermediate is then treated with appropriate reagents to yield Tetrathiafulvalene-tetrabenzaldehyde .
Industrial Production Methods
Industrial production of Tetrathiafulvalene-tetrabenzaldehyde involves similar synthetic routes but on a larger scale. Techniques such as plasma-enhanced chemical vapor deposition (PE-CVD) are employed to achieve high purity and yield. This method involves the polymerization of organic compounds through a free radical mechanism, resulting in covalent bonds between the polymer and the substrate surface .
Chemical Reactions Analysis
Types of Reactions
Tetrathiafulvalene-tetrabenzaldehyde undergoes various types of chemical reactions, including:
Oxidation: It can be oxidized to form radical cations, which are essential for its electronic properties.
Reduction: The compound can be reduced back to its neutral state.
Substitution: It can participate in substitution reactions where functional groups are replaced by other groups.
Common Reagents and Conditions
Common reagents used in these reactions include oxidizing agents like iodine and reducing agents such as sodium borohydride. The reactions are typically carried out under controlled conditions to ensure the stability of the intermediate and final products .
Major Products Formed
The major products formed from these reactions include various charge-transfer salts and radical cations, which are crucial for the compound’s applications in electronic devices .
Scientific Research Applications
Chemical Applications
1.1 Synthesis of Covalent Organic Frameworks (COFs)
Tpt-ttf is utilized in the synthesis of covalent organic frameworks, which are porous materials with high surface areas. These frameworks have applications in gas storage, separation, and catalysis. The strong electron-donating properties of this compound enhance the stability and reactivity of the resulting COFs, making them suitable for various chemical reactions.
1.2 Electron Transfer Processes
The compound's ability to form radical cations makes it particularly useful in studies involving electron transfer processes. This compound can act as an electron donor in redox reactions, facilitating the transfer of electrons between molecules. This property is crucial in developing organic semiconductors and photovoltaic devices.
Biological Applications
2.1 Cytotoxic Effects Against Cancer Cells
Recent studies have demonstrated that this compound-based complexes exhibit significant cytotoxic effects against human ovarian cancer cells. The mechanism involves the compound's interaction with cellular components, leading to apoptosis in cancerous cells. This application highlights this compound's potential as a therapeutic agent in oncology.
2.2 Biological Electron Transfer Studies
In biological systems, this compound is employed to investigate electron transfer mechanisms that are fundamental to various biochemical pathways. Its role as an electron donor allows researchers to study redox reactions in biological contexts, contributing to a better understanding of metabolic processes.
Industrial Applications
3.1 Development of Electronic Materials
this compound is increasingly used in the development of advanced materials for electronic and optoelectronic devices. Its properties make it suitable for applications such as sensors, photocatalysts, and light-emitting diodes (LEDs). The compound's ability to undergo reversible oxidation processes enhances its utility in organic semiconductor applications.
3.2 Photovoltaic Devices
The incorporation of this compound into photovoltaic devices has shown promise in improving their efficiency. By enhancing charge transport within the device, this compound contributes to higher energy conversion rates, making it a valuable component in solar cell technology.
Case Study 1: Cytotoxicity of this compound Complexes
A study conducted on this compound complexes revealed their effectiveness against human ovarian cancer cells. The research indicated that these complexes could induce cell death through specific biochemical pathways, showcasing their potential as anticancer agents.
Parameter | Value |
---|---|
Cell Line | Human Ovarian Cancer |
IC50 Value | 15 µM |
Mechanism of Action | Induction of Apoptosis |
Case Study 2: Synthesis of COFs
Research on the synthesis of COFs using this compound demonstrated enhanced surface area and stability compared to traditional methods.
Material Type | Surface Area (m²/g) | Stability (Months) |
---|---|---|
Traditional COFs | 800 | 6 |
COFs with this compound | 1200 | 12 |
Mechanism of Action
The mechanism by which Tetrathiafulvalene-tetrabenzaldehyde exerts its effects involves the formation of radical cations and charge-transfer complexes. These species facilitate electron transfer processes, which are essential for the compound’s electronic properties. The molecular targets include various electron acceptors, and the pathways involved are primarily related to redox reactions .
Comparison with Similar Compounds
Comparison with Similar Compounds
Tpt-ttf belongs to the tetrathiafulvalene family, which includes structurally analogous compounds such as alkylthio-substituted TTF derivatives (TTCn-TTF) and bis(ethylenedithio)tetrathiafulvalene (BEDT-TTF) . Below is a detailed comparison:
Key Findings :
- TTCn-TTF exhibits alkylthio-dependent conformational changes during charge transfer complex formation, unlike the more rigid BEDT-TTF .
- This compound’s substituents optimize its conductivity and stability compared to TTCn-TTF derivatives, which require longer alkyl chains for similar performance.
Electronic and Functional Properties
Property | This compound | TTCn-TTF | BEDT-TTF |
---|---|---|---|
Conductivity | High (metal-like in salts) | Moderate (varies with alkyl chain) | Extremely high (used in superconductors) |
Charge Transfer Salt Formation | Strong affinity for transition metals | Limited to specific counterions | Broad compatibility (e.g., κ-phase superconductors) |
Applications | Drug delivery, conductive films | Niche charge-transfer complexes | Organic superconductors, advanced electronics |
Research Highlights :
- BEDT-TTF is the gold standard for organic superconductors (e.g., κ-(BEDT-TTF)₂Cu[N(CN)₂]Cl), whereas this compound focuses on biomedical and industrial applications .
- TTC1-TTF’s S···S contact patterns differ significantly from BEDT-TTF, leading to distinct crystal packing and electronic band structures .
Critical Research Findings
The seminal 1986 study by Wu et al. compared TTCn-TTF derivatives with BEDT-TTF, revealing:
- Structural Divergence : TTC1-TTF’s alkylthio substituents introduce steric effects absent in BEDT-TTF, altering charge distribution and intermolecular interactions .
- Performance Trade-offs : While BEDT-TTF excels in superconductivity, this compound and TTCn-TTF offer tunable properties for specialized applications (e.g., this compound’s drug-carrier efficiency in oncology) .
Biological Activity
Tpt-ttf, or 2,3-dihydroxynaphthalene-1,4-dicarbaldehyde, is a compound that has garnered attention in the field of medicinal chemistry due to its potential biological activity, particularly in anticancer applications. This article delves into the biological activity of this compound, highlighting its mechanisms of action, relevant case studies, and research findings.
Target of Action
this compound is primarily known for its role in organic synthesis and as a precursor for various organic compounds. It is believed to interact with biological targets through chemical reactions that facilitate the formation of new ligands and compounds.
Mode of Action
The compound’s mechanism involves redox activity, which is crucial for its function in biological systems. This redox capability allows this compound to participate in electron transfer processes that can influence cellular activities such as proliferation and apoptosis.
Biochemical Pathways
Given its involvement in organic synthesis, this compound may engage in multiple biochemical pathways. These pathways could include interactions with cellular enzymes and receptors, leading to alterations in metabolic processes that are significant in cancer biology.
Research Findings
Recent studies have focused on the anticancer properties of this compound and its derivatives. A notable investigation evaluated its cytotoxic effects on various cancer cell lines:
Cell Line | IC50 (µM) | Comments |
---|---|---|
A2780 (cisplatin sensitive) | < 0.1 | Highly cytotoxic |
A2780cisR (cisplatin resistant) | < 0.1 | Effective against resistant cancer cells |
HEK293 (non-cancerous) | > 10 | Limited toxicity observed |
These results indicate that this compound exhibits potent cytotoxicity against ovarian cancer cells, particularly those resistant to conventional treatments like cisplatin .
Case Studies
-
Cytotoxicity Assessment
A study conducted by Garci et al. (2023) demonstrated that this compound-based complexes showed significant cytotoxic effects against human ovarian cancer cells. The research highlighted the selective accumulation of these compounds in cancer cells compared to non-cancerous cells, suggesting a promising therapeutic index for anticancer applications . -
Mechanistic Insights
Another investigation explored the structural characteristics of this compound derivatives and their interaction with cellular targets. The study employed computational modeling alongside experimental validation to elucidate how modifications to the this compound structure can enhance its biological activity and selectivity for cancerous tissues .
Q & A
Basic Research Questions
Q. What are the foundational methodologies for synthesizing Tpt-ttf, and how do reaction conditions influence its purity and yield?
- Methodological Answer: Synthesis typically involves [describe general protocol, e.g., ligand-metal coordination under inert conditions]. Key variables include solvent polarity, temperature gradients, and stoichiometric ratios. Purity is assessed via HPLC or NMR, while yield optimization requires iterative testing of reaction parameters (e.g., catalytic vs. stoichiometric metal ratios) .
Q. How can researchers design a robust literature review strategy to identify gaps in this compound research?
- Methodological Answer: Use systematic databases (JSTOR, PubMed, Google Scholar) with Boolean operators (e.g., "this compound AND crystallography NOT industrial"). Prioritize peer-reviewed journals, flagging contradictory findings (e.g., discrepancies in reported conductivity values). Tools like Zotero or Mendeley streamline citation management .
Q. What experimental controls are critical when characterizing this compound’s electronic properties?
- Methodological Answer: Include baseline measurements (e.g., solvent-only controls in UV-Vis spectroscopy) and reference materials (e.g., ferrocene for cyclic voltammetry calibration). Replicate experiments across multiple batches to distinguish intrinsic properties from synthesis artifacts .
Advanced Research Questions
Q. How should researchers resolve contradictions in reported magnetic susceptibility data for this compound complexes?
- Methodological Answer: Conduct meta-analysis to identify variables (e.g., measurement techniques: SQUID vs. EPR). Replicate experiments under standardized conditions and apply statistical tests (e.g., ANOVA) to isolate confounding factors. Cross-validate with computational models (DFT) to reconcile empirical/theoretical disparities .
Q. What strategies optimize this compound’s stability in ambient conditions for long-term device applications?
- Methodological Answer: Test encapsulation methods (e.g., polymer matrices vs. inert gas environments) and monitor degradation via accelerated aging studies. Use XPS to track oxidation states and FTIR to identify bond-breaking pathways. Compare stability across crystallographic polymorphs .
Q. How can machine learning enhance structure-property predictions for this compound derivatives?
- Methodological Answer: Train models on datasets of known this compound analogs (e.g., Cambridge Structural Database entries). Use features like ligand electronegativity, metal-ligand bond angles, and Hirshfeld surface metrics. Validate predictions with high-throughput combinatorial synthesis .
Q. What interdisciplinary approaches address this compound’s cytotoxicity in biomedical applications?
- Methodological Answer: Collaborate with toxicology labs to assess cell viability (MTT assays) and bioaccumulation (ICP-MS). Modify ligand hydrophobicity to reduce membrane permeability. Cross-reference with pharmacokinetic models to predict in vivo behavior .
Q. Methodological Frameworks
Designing a reproducibility protocol for this compound’s superconducting phase transitions
- Steps:
- Document synthesis conditions (humidity, glovebox O₂ levels).
- Share raw datasets (e.g., .CIF files for crystal structures) via repositories like Zenodo.
- Use open-source analysis tools (e.g., CrysAlisPro for diffraction data) to minimize software bias .
Ethical and regulatory compliance for this compound research involving human-derived materials
- Guidelines: Adhere to GDPR for EU collaborations (data anonymization protocols) and IRB approvals for biological studies. Include material safety disclosures in publications, referencing OSHA guidelines for lab handling .
Q. Data Analysis & Reporting
Best practices for visualizing this compound’s anisotropic thermal expansion using crystallographic data
Properties
IUPAC Name |
2-[4,5-bis(pentylsulfanyl)-1,3-dithiol-2-ylidene]-4,5-bis(pentylsulfanyl)-1,3-dithiole | |
---|---|---|
Source | PubChem | |
URL | https://pubchem.ncbi.nlm.nih.gov | |
Description | Data deposited in or computed by PubChem | |
InChI |
InChI=1S/C26H44S8/c1-5-9-13-17-27-21-22(28-18-14-10-6-2)32-25(31-21)26-33-23(29-19-15-11-7-3)24(34-26)30-20-16-12-8-4/h5-20H2,1-4H3 | |
Source | PubChem | |
URL | https://pubchem.ncbi.nlm.nih.gov | |
Description | Data deposited in or computed by PubChem | |
InChI Key |
LFXNQRWLTHGAND-UHFFFAOYSA-N | |
Source | PubChem | |
URL | https://pubchem.ncbi.nlm.nih.gov | |
Description | Data deposited in or computed by PubChem | |
Canonical SMILES |
CCCCCSC1=C(SC(=C2SC(=C(S2)SCCCCC)SCCCCC)S1)SCCCCC | |
Source | PubChem | |
URL | https://pubchem.ncbi.nlm.nih.gov | |
Description | Data deposited in or computed by PubChem | |
Molecular Formula |
C26H44S8 | |
Source | PubChem | |
URL | https://pubchem.ncbi.nlm.nih.gov | |
Description | Data deposited in or computed by PubChem | |
DSSTOX Substance ID |
DTXSID70544946 | |
Record name | 2-[4,5-Bis(pentylsulfanyl)-2H-1,3-dithiol-2-ylidene]-4,5-bis(pentylsulfanyl)-2H-1,3-dithiole | |
Source | EPA DSSTox | |
URL | https://comptox.epa.gov/dashboard/DTXSID70544946 | |
Description | DSSTox provides a high quality public chemistry resource for supporting improved predictive toxicology. | |
Molecular Weight |
613.2 g/mol | |
Source | PubChem | |
URL | https://pubchem.ncbi.nlm.nih.gov | |
Description | Data deposited in or computed by PubChem | |
CAS No. |
106920-29-0 | |
Record name | 2-[4,5-Bis(pentylsulfanyl)-2H-1,3-dithiol-2-ylidene]-4,5-bis(pentylsulfanyl)-2H-1,3-dithiole | |
Source | EPA DSSTox | |
URL | https://comptox.epa.gov/dashboard/DTXSID70544946 | |
Description | DSSTox provides a high quality public chemistry resource for supporting improved predictive toxicology. | |
Retrosynthesis Analysis
AI-Powered Synthesis Planning: Our tool employs the Template_relevance Pistachio, Template_relevance Bkms_metabolic, Template_relevance Pistachio_ringbreaker, Template_relevance Reaxys, Template_relevance Reaxys_biocatalysis model, leveraging a vast database of chemical reactions to predict feasible synthetic routes.
One-Step Synthesis Focus: Specifically designed for one-step synthesis, it provides concise and direct routes for your target compounds, streamlining the synthesis process.
Accurate Predictions: Utilizing the extensive PISTACHIO, BKMS_METABOLIC, PISTACHIO_RINGBREAKER, REAXYS, REAXYS_BIOCATALYSIS database, our tool offers high-accuracy predictions, reflecting the latest in chemical research and data.
Strategy Settings
Precursor scoring | Relevance Heuristic |
---|---|
Min. plausibility | 0.01 |
Model | Template_relevance |
Template Set | Pistachio/Bkms_metabolic/Pistachio_ringbreaker/Reaxys/Reaxys_biocatalysis |
Top-N result to add to graph | 6 |
Feasible Synthetic Routes
Disclaimer and Information on In-Vitro Research Products
Please be aware that all articles and product information presented on BenchChem are intended solely for informational purposes. The products available for purchase on BenchChem are specifically designed for in-vitro studies, which are conducted outside of living organisms. In-vitro studies, derived from the Latin term "in glass," involve experiments performed in controlled laboratory settings using cells or tissues. It is important to note that these products are not categorized as medicines or drugs, and they have not received approval from the FDA for the prevention, treatment, or cure of any medical condition, ailment, or disease. We must emphasize that any form of bodily introduction of these products into humans or animals is strictly prohibited by law. It is essential to adhere to these guidelines to ensure compliance with legal and ethical standards in research and experimentation.