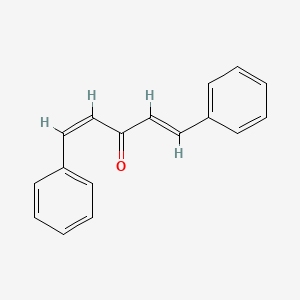
1,5-Diphenylpenta-1,4-dien-3-one
Overview
Description
1,5-Diphenylpenta-1,4-dien-3-one, also known as dibenzylideneacetone, is an organic compound with the molecular formula C₁₇H₁₄O. It is a yellow crystalline solid that is soluble in organic solvents. This compound is notable for its conjugated system, which imparts unique chemical and physical properties. It is used in various fields, including organic synthesis, material science, and as a ligand in coordination chemistry .
Preparation Methods
Synthetic Routes and Reaction Conditions
1,5-Diphenylpenta-1,4-dien-3-one is typically synthesized through the aldol condensation reaction between benzalacetone and benzaldehyde. The reaction is carried out in the presence of a strong base, such as sodium hydroxide or potassium hydroxide, under reflux conditions. The general reaction scheme is as follows:
C6H5CH=CHCOCH3+C6H5CHO→C6H5CH=CHCOCH=CHC6H5
The product is then purified by recrystallization from an appropriate solvent .
Industrial Production Methods
In industrial settings, the synthesis of this compound follows similar principles but is scaled up to accommodate larger production volumes. The reaction conditions are optimized to maximize yield and purity, often involving continuous flow reactors and automated systems to control temperature and reagent addition .
Chemical Reactions Analysis
Types of Reactions
1,5-Diphenylpenta-1,4-dien-3-one undergoes various chemical reactions, including:
Oxidation: The compound can be oxidized to form corresponding diketones.
Reduction: Reduction reactions can convert it into saturated ketones.
Substitution: Electrophilic substitution reactions can introduce different functional groups onto the phenyl rings
Common Reagents and Conditions
Oxidation: Common oxidizing agents include potassium permanganate and chromium trioxide.
Reduction: Reducing agents such as sodium borohydride and lithium aluminum hydride are used.
Substitution: Reagents like halogens, nitrating agents, and sulfonating agents are employed under controlled conditions
Major Products
Oxidation: Produces diketones.
Reduction: Yields saturated ketones.
Substitution: Results in various substituted derivatives depending on the reagents used
Scientific Research Applications
1,5-Diphenylpenta-1,4-dien-3-one has several scientific research applications:
Chemistry: Used as a ligand in coordination chemistry and as a starting material for synthesizing more complex molecules.
Biology: Investigated for its potential antioxidant properties and ability to scavenge free radicals.
Medicine: Studied for its potential anticancer properties and as a component in drug formulations.
Industry: Utilized in the production of polymers, coatings, and as a corrosion inhibitor .
Mechanism of Action
The mechanism of action of 1,5-diphenylpenta-1,4-dien-3-one involves its ability to interact with various molecular targets. For example, its antioxidant activity is attributed to its ability to donate electrons to neutralize free radicals. In coordination chemistry, it acts as a ligand, forming complexes with metal ions through its carbonyl and conjugated double bonds .
Comparison with Similar Compounds
Similar Compounds
Benzalacetone: A precursor in the synthesis of 1,5-diphenylpenta-1,4-dien-3-one.
Benzaldehyde: Another precursor used in the synthesis.
Dibenzylideneacetone: A stereoisomer with similar properties
Uniqueness
This compound is unique due to its extended conjugated system, which imparts distinct electronic properties. This makes it particularly useful in applications requiring specific optical and electronic characteristics .
Properties
CAS No. |
115587-57-0 |
---|---|
Molecular Formula |
C17H14O |
Molecular Weight |
234.29 g/mol |
IUPAC Name |
(1Z,4E)-1,5-diphenylpenta-1,4-dien-3-one |
InChI |
InChI=1S/C17H14O/c18-17(13-11-15-7-3-1-4-8-15)14-12-16-9-5-2-6-10-16/h1-14H/b13-11-,14-12+ |
InChI Key |
WMKGGPCROCCUDY-HEEUSZRZSA-N |
SMILES |
C1=CC=C(C=C1)C=CC(=O)C=CC2=CC=CC=C2 |
Isomeric SMILES |
C1=CC=C(C=C1)/C=C/C(=O)/C=C\C2=CC=CC=C2 |
Canonical SMILES |
C1=CC=C(C=C1)C=CC(=O)C=CC2=CC=CC=C2 |
Origin of Product |
United States |
Synthesis routes and methods I
Procedure details
Synthesis routes and methods II
Procedure details
Synthesis routes and methods III
Procedure details
Retrosynthesis Analysis
AI-Powered Synthesis Planning: Our tool employs the Template_relevance Pistachio, Template_relevance Bkms_metabolic, Template_relevance Pistachio_ringbreaker, Template_relevance Reaxys, Template_relevance Reaxys_biocatalysis model, leveraging a vast database of chemical reactions to predict feasible synthetic routes.
One-Step Synthesis Focus: Specifically designed for one-step synthesis, it provides concise and direct routes for your target compounds, streamlining the synthesis process.
Accurate Predictions: Utilizing the extensive PISTACHIO, BKMS_METABOLIC, PISTACHIO_RINGBREAKER, REAXYS, REAXYS_BIOCATALYSIS database, our tool offers high-accuracy predictions, reflecting the latest in chemical research and data.
Strategy Settings
Precursor scoring | Relevance Heuristic |
---|---|
Min. plausibility | 0.01 |
Model | Template_relevance |
Template Set | Pistachio/Bkms_metabolic/Pistachio_ringbreaker/Reaxys/Reaxys_biocatalysis |
Top-N result to add to graph | 6 |
Feasible Synthetic Routes
Disclaimer and Information on In-Vitro Research Products
Please be aware that all articles and product information presented on BenchChem are intended solely for informational purposes. The products available for purchase on BenchChem are specifically designed for in-vitro studies, which are conducted outside of living organisms. In-vitro studies, derived from the Latin term "in glass," involve experiments performed in controlled laboratory settings using cells or tissues. It is important to note that these products are not categorized as medicines or drugs, and they have not received approval from the FDA for the prevention, treatment, or cure of any medical condition, ailment, or disease. We must emphasize that any form of bodily introduction of these products into humans or animals is strictly prohibited by law. It is essential to adhere to these guidelines to ensure compliance with legal and ethical standards in research and experimentation.