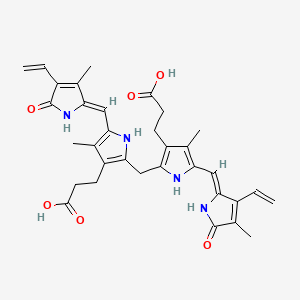
Bilirubin
Overview
Description
Bilirubin is a tetrapyrrole compound produced during heme catabolism, primarily derived from the breakdown of hemoglobin in senescent red blood cells. It exists in two forms: unconjugated (indirect) this compound, which is lipid-soluble and transported to the liver bound to albumin, and conjugated (direct) this compound, which is water-soluble and excreted into bile . Elevated levels of unconjugated this compound are associated with neurotoxicity, particularly in neonates, due to its ability to cross the blood-brain barrier and induce oxidative stress . Conversely, this compound exhibits antioxidant and anti-inflammatory properties, with studies suggesting protective roles against metabolic syndrome (MetS), diabetes, and cardiovascular diseases .
Preparation Methods
Synthetic Routes and Reaction Conditions: Bilirubin can be produced via whole-cell transformation utilizing recombinant Corynebacterium glutamicum expressing a β-glucuronidase from Staphylococcus sp. RLH1. This method involves hydrolyzing conjugated this compound to this compound under optimized biotransformation conditions, achieving a conversion rate of 79.7% at 40°C, pH 7.0, 1 mM Mg²⁺, and 6 mM antioxidant NaHSO₃ after 12 hours .
Industrial Production Methods: Industrial production of this compound typically involves extracting it from mammalian bile. The existing production methods use alkaline hydrolysis to remove the β-glucuronic acid group from conjugated this compound, employing a highly concentrated sodium hydroxide solution at high temperature . Another method involves the biotransformation of biliverdin using recombinant Escherichia coli cells expressing biliverdin reductase, achieving a conversion yield of 72.3% .
Chemical Reactions Analysis
Types of Reactions: Bilirubin undergoes various chemical reactions, including oxidation, reduction, and conjugation. For instance, this compound may be oxidized by a chemical compound (vanadate) or the enzyme this compound oxidase to biliverdin, which subsequently oxidizes to initially purple and then colorless products .
Common Reagents and Conditions:
Oxidation: Vanadate or this compound oxidase.
Reduction: Biliverdin reductase with NADPH as the reducing agent.
Conjugation: Glucuronic acid added to this compound by the enzyme glucuronyl transferase.
Major Products:
Oxidation: Biliverdin.
Reduction: this compound from biliverdin.
Conjugation: Conjugated this compound (this compound diglucuronide).
Scientific Research Applications
Antioxidant Properties
Bilirubin is recognized for its potent antioxidant capabilities. It plays a crucial role in protecting cells from oxidative stress, which is implicated in numerous diseases.
- Case Study: Neuroprotection
In a study conducted by Johns Hopkins Medicine, researchers discovered that this compound levels in mouse brains are significantly higher than in the liver. This suggests a protective role against oxidative stress in neurodegenerative diseases like Parkinson's and Huntington's. The study found that this compound may help shield brain cells from damage caused by oxidative stress, indicating its potential as a therapeutic agent for neuroprotection .
Cardiovascular Health
This compound has been linked to cardiovascular benefits, particularly in reducing ischemia-reperfusion injury.
- Case Study: Ischemia-Reperfusion Injury
Experimental models demonstrated that administering this compound prior to inducing ischemia resulted in decreased infarct size and improved cardiac function. For instance, Gunn rats with congenital hyperbilirubinemia showed enhanced coronary flow and reduced damage during ischemic events .
Metabolic Disorders
Recent studies indicate that this compound may have beneficial effects on metabolic disorders such as obesity and diabetes.
- Case Study: Insulin Sensitivity
Research involving diet-induced obesity (DIO) mouse models showed that this compound treatment led to significant reductions in body weight, blood glucose levels, and cholesterol. The treated mice exhibited improved insulin sensitivity and favorable changes in adipokine levels, suggesting this compound's potential role in managing metabolic syndrome .
Pulmonary Health
This compound's anti-inflammatory properties extend to respiratory conditions.
- Case Study: Smoke-Induced Emphysema
In a rat model of smoke-induced emphysema, this compound administration suppressed pulmonary inflammation and oxidative damage. The treatment resulted in reduced leukocyte accumulation and pro-inflammatory cytokine levels, highlighting this compound's protective effects against lung injury .
Renal Protection
Emerging evidence suggests that this compound may offer protective benefits for kidney health.
- Case Study: Chronic Kidney Disease
A study involving over 1,000 patients indicated that mildly elevated serum this compound levels were associated with better renal outcomes. This compound treatment reduced renal fibrosis in animal models, suggesting its potential as a therapeutic target for delaying kidney disease progression .
Clinical Applications and Challenges
While the therapeutic potential of this compound is promising, several challenges hinder its clinical application:
- Solubility Issues : this compound is poorly soluble in water, complicating its formulation as a drug.
- Source Concerns : Current sources of this compound are often animal-derived, raising concerns about safety.
- Regulatory Considerations : Maintaining mild elevations of this compound for therapeutic purposes could lead to iatrogenic conditions similar to Gilbert’s Syndrome .
Data Summary Table
Mechanism of Action
Bilirubin exerts its effects through various mechanisms:
Antioxidant Activity: this compound acts as a powerful lipid-soluble antioxidant, scavenging reactive oxygen species (ROS) in the body.
Metabolic Hormone: Recent research suggests that this compound functions as a metabolic hormone, driving gene transcription by nuclear receptors.
Immunomodulatory Effects: this compound decreases the release of damage-associated molecular patterns (DAMPs) and interleukin-1β (IL-1β) by injured cells, reducing inflammation.
Comparison with Similar Compounds
Comparison with Structurally and Functionally Similar Compounds
Biliverdin
Biliverdin, the immediate precursor to this compound, shares a tetrapyrrole structure but differs in oxidation state. In a study evaluating MIP specificity, biliverdin reduced this compound binding capacity by 15–20% in binary mixtures, highlighting structural similarities that allow partial competition. However, MIPs retained preferential affinity for this compound, emphasizing subtle conformational distinctions .
Phycobilin Analogues
Phycobilin analogues, designed to mimic this compound’s tetrapyrrole core, demonstrate enhanced excitonic coupling in spectroscopic studies. Computational models revealed that altering bridge groups (e.g., methyl or phenyl substitutions) increased coupling energy by 30–40% compared to native this compound, enabling tailored photophysical properties for optoelectronic applications .
Conjugated vs. Unconjugated this compound
While both forms derive from heme metabolism, their solubility and bioactivity differ markedly. Conjugated this compound’s hydrophilic nature prevents neurotoxicity but facilitates biliary excretion. Automated assays distinguish these forms without cross-reactivity, critical for diagnosing hepatic dysfunction .
Hyaluronic Acid-Bilirubin Nanoparticles (HABNs)
HABNs combine this compound’s anti-inflammatory effects with hyaluronic acid’s targeting capacity, illustrating how this compound’s chemical properties can be harnessed in nanomedicine despite structural modifications .
Mechanistic Insights and Clinical Implications
- Transport Specificity: this compound is transported by hepatic Organic Anion Transporting Polypeptides (OATPs), which also handle bile salts and hormones.
- Diagnostic Interference: this compound analogues like biliverdin and steroids (progesterone, testosterone) show minimal cross-reactivity in immunoassays, ensuring accurate clinical quantification .
Biological Activity
Bilirubin, a bile pigment resulting from the breakdown of heme, has traditionally been regarded as a waste product. However, recent research has illuminated its multifaceted biological activities, particularly its roles as an antioxidant and immunomodulatory agent. This article delves into the biological activity of this compound, highlighting its physiological significance, mechanisms of action, and implications for health.
Antioxidant Properties
This compound is well-established as a potent antioxidant . Its antioxidant activity is notably enhanced under low oxygen conditions, such as those found in tissues (approximately 2% oxygen) compared to atmospheric levels (20%). This property allows this compound to scavenge free radicals effectively, thereby protecting cells from oxidative stress .
Mechanisms of Antioxidant Action
- Scavenging Free Radicals : this compound can neutralize reactive oxygen species (ROS), reducing cellular damage.
- Regulating Antioxidant Enzymes : It influences the expression of various antioxidant enzymes, enhancing the body's overall antioxidant capacity.
Immunomodulatory Effects
Recent studies have revealed that this compound possesses significant immunomodulatory properties . It has been shown to inhibit T cell responses, suggesting a role in immune tolerance .
Key Findings on Immunomodulation
- Inhibition of T Cell Activation : this compound suppresses both antigen-specific and polyclonal T cell responses, which may be beneficial in preventing autoimmune diseases .
- Induction of Immune Tolerance : this compound promotes immune tolerance in various experimental settings, indicating potential therapeutic applications in transplantation and autoimmune disorders .
Metabolic Regulation
This compound has been identified as a potential metabolic hormone , interacting with nuclear receptors such as PPARα (Peroxisome Proliferator-Activated Receptor Alpha). This interaction plays a crucial role in regulating lipid metabolism and reducing fat accumulation in the liver .
Implications for Metabolic Health
- Obesity Management : By modulating PPARα activity, this compound may help reduce obesity and improve metabolic dysfunctions .
- Cardiovascular Health : Elevated this compound levels are associated with decreased cardiovascular risk, particularly in men .
Table 1: Summary of this compound's Biological Activities
Activity Type | Mechanism/Effect | Implications |
---|---|---|
Antioxidant | Scavenges free radicals | Protects against oxidative stress |
Immunomodulatory | Inhibits T cell activation | Potential therapeutic use in autoimmune diseases |
Metabolic Regulation | Interacts with PPARα | Reduces liver fat accumulation |
Cardiovascular Protection | Correlates with lower cardiovascular risk | Possible protective role against heart disease |
Notable Research Studies
- Immunomodulatory Study : A study demonstrated that this compound significantly inhibits T cell activation and induces immune tolerance both in vitro and in vivo, suggesting its potential use in clinical settings to modulate immune responses .
- Metabolic Hormone Investigation : Research indicated that this compound acts on PPARα to regulate lipid metabolism, with experiments showing reduced fat accumulation in genetically modified mice lacking PPARα .
- Cardiovascular Risk Assessment : A longitudinal study found that individuals with moderately elevated this compound levels exhibited a lower incidence of metabolic syndrome and cardiovascular events .
Q & A
Basic Research Questions
Q. What standardized methodologies are recommended for accurate bilirubin quantification in experimental settings?
The Jendrassik-Grof method is widely validated for this compound assays due to its precision (CV <0.5%) and consistency across protein matrices like human serum albumin (HSA) and bovine serum albumin (BSA). However, standardization challenges persist, as molar absorptivity varies in methods like Malloy-Evelyn when using serum vs. synthetic matrices . Researchers should calibrate instruments using stable this compound standards stored at -70°C to minimize degradation .
Q. How does this compound's antioxidant activity influence experimental design in oxidative stress studies?
this compound's role as a cytoprotectant involves scavenging reactive oxygen species (ROS) and modulating cellular signaling pathways (e.g., via biliverdin reductase). Experimental designs should include controls for endogenous this compound levels and use assays like thiobarbituric acid-reactive substances (TBARS) to quantify lipid peroxidation. Dose-response studies must account for this compound's U-shaped toxicity curve, where both deficiency and excess can confound results .
Q. What are the critical parameters for reproducing this compound-related synthesis and characterization in vitro?
For novel this compound derivatives, provide NMR, HPLC, and mass spectrometry data to confirm purity (>95%) and structural identity. Report reaction conditions (solvent, temperature, catalysts) and yields, prioritizing gravimetric measurements over spectroscopic estimates. For known compounds, cross-reference literature melting points and spectral data to ensure reproducibility .
Advanced Research Questions
Q. How can contradictory epidemiological data on this compound's atheroprotective effects be resolved?
The NHANES study (1999–2004) found a 6% reduction in peripheral arterial disease (PAD) odds per 0.1 mg/dL this compound increase, but effect sizes varied by gender (OR 0.90 in men vs. 0.97 in women) and smoking status (OR 0.81 in smokers). To address contradictions, stratify analyses by covariates like smoking and gender, and use Mendelian randomization to mitigate confounding from reverse causation .
Q. What experimental frameworks validate this compound's therapeutic potential in neurodegenerative diseases?
Preclinical models (e.g., Alzheimer’s transgenic mice) should incorporate this compound administration via controlled-release nanoparticles to maintain physiological concentrations. Assess outcomes using behavioral assays (e.g., Morris water maze) and biomarkers like amyloid-β40/42. Compare results against known antioxidants (e.g., vitamin E) to isolate this compound-specific effects .
Q. How do neonatal this compound dynamics inform the design of pediatric pharmacokinetic studies?
In ABO hemolytic disease, this compound peaks at 72–96 hours postpartum. Retrospective cohort designs (e.g., n=235 neonates) should correlate total this compound (TBIL) and indirect this compound (IBIL) with clinic day age, adjusting for phototherapy duration and maternal IgG titers. Use nonlinear mixed-effects modeling (NONMEM) to account for age-dependent metabolism .
Q. Methodological Challenges
Q. What statistical approaches address heteroscedasticity in this compound-clinical outcome associations?
Apply log-transformation to this compound levels to normalize skewed distributions. For outcomes like PAD, use multivariable logistic regression with bootstrapping (1,000 iterations) to estimate confidence intervals. Sensitivity analyses should exclude outliers (e.g., Gilbert’s syndrome patients) to test robustness .
Q. How can this compound’s labile nature be managed in longitudinal cohort studies?
Collect serum samples in amber vials with EDTA to prevent oxidation. Store at -80°C and avoid freeze-thaw cycles. For degraded samples, quantify this compound degradation products (e.g., biliverdin) via high-performance liquid chromatography (HPLC) and adjust analyses using correction factors .
Q. Emerging Research Directions
Q. What novel biomarkers integrate this compound with omics data for early disease detection?
Combine this compound levels with proteomic signatures (e.g., haptoglobin isoforms) and genomic variants (e.g., UGT1A1 polymorphisms) in machine learning models. Validate predictive accuracy using ROC curves in multi-ethnic cohorts to ensure generalizability .
Q. How can CRISPR-Cas9 models elucidate this compound’s role in circadian regulation?
Generate hepatocyte-specific UGT1A1 knockout mice to study hyperbilirubinemia’s effects on circadian genes (e.g., CLOCK, BMAL1). Use luciferase reporters to monitor real-time gene expression rhythms under controlled light-dark cycles .
Q. Ethical and Reporting Standards
Q. What ethical considerations apply to this compound trials involving pediatric populations?
Obtain informed consent from guardians and assent from children >7 years. Monitor for kernicterus risk via auditory brainstem response (ABR) testing in neonates. Report adverse events (e.g., exchange transfusions) per CONSORT guidelines .
Q. How should conflicting this compound data be reported to enhance reproducibility?
Disclose assay methodologies (e.g., diazo vs. enzymatic), calibration standards, and inter-laboratory CVs. Use supplemental tables to list raw data and analytical code repositories (e.g., GitHub) for transparency .
Properties
CAS No. |
93891-87-3 |
---|---|
Molecular Formula |
C33H34N4Na2O6 |
Molecular Weight |
628.6 g/mol |
IUPAC Name |
disodium;3-[2-[[3-(2-carboxylatoethyl)-5-[(Z)-(3-ethenyl-4-methyl-5-oxopyrrol-2-ylidene)methyl]-4-methyl-1H-pyrrol-2-yl]methyl]-5-[(Z)-(4-ethenyl-3-methyl-5-oxopyrrol-2-ylidene)methyl]-4-methyl-1H-pyrrol-3-yl]propanoate |
InChI |
InChI=1S/C33H36N4O6.2Na/c1-7-20-19(6)32(42)37-27(20)14-25-18(5)23(10-12-31(40)41)29(35-25)15-28-22(9-11-30(38)39)17(4)24(34-28)13-26-16(3)21(8-2)33(43)36-26;;/h7-8,13-14,34-35H,1-2,9-12,15H2,3-6H3,(H,36,43)(H,37,42)(H,38,39)(H,40,41);;/q;2*+1/p-2/b26-13-,27-14-;; |
InChI Key |
XNHLFNMMORFXAO-PDQJREFDSA-L |
SMILES |
CC1=C(NC(=C1CCC(=O)O)CC2=C(C(=C(N2)C=C3C(=C(C(=O)N3)C)C=C)C)CCC(=O)O)C=C4C(=C(C(=O)N4)C=C)C |
Isomeric SMILES |
CC1=C(NC(=C1CCC(=O)[O-])CC2=C(C(=C(N2)/C=C\3/C(=C(C(=O)N3)C)C=C)C)CCC(=O)[O-])/C=C\4/C(=C(C(=O)N4)C=C)C.[Na+].[Na+] |
Canonical SMILES |
CC1=C(NC(=C1CCC(=O)[O-])CC2=C(C(=C(N2)C=C3C(=C(C(=O)N3)C)C=C)C)CCC(=O)[O-])C=C4C(=C(C(=O)N4)C=C)C.[Na+].[Na+] |
physical_description |
Light orange to reddish-brown solid; [Merck Index] Red powder; [Sigma-Aldrich MSDS] Solid |
Related CAS |
18422-02-1 (calcium salt) 93891-87-3 (di-hydrochloride salt) 635-65-4 (Parent) |
solubility |
0.009 mg/mL at 25 °C |
Origin of Product |
United States |
Retrosynthesis Analysis
AI-Powered Synthesis Planning: Our tool employs the Template_relevance Pistachio, Template_relevance Bkms_metabolic, Template_relevance Pistachio_ringbreaker, Template_relevance Reaxys, Template_relevance Reaxys_biocatalysis model, leveraging a vast database of chemical reactions to predict feasible synthetic routes.
One-Step Synthesis Focus: Specifically designed for one-step synthesis, it provides concise and direct routes for your target compounds, streamlining the synthesis process.
Accurate Predictions: Utilizing the extensive PISTACHIO, BKMS_METABOLIC, PISTACHIO_RINGBREAKER, REAXYS, REAXYS_BIOCATALYSIS database, our tool offers high-accuracy predictions, reflecting the latest in chemical research and data.
Strategy Settings
Precursor scoring | Relevance Heuristic |
---|---|
Min. plausibility | 0.01 |
Model | Template_relevance |
Template Set | Pistachio/Bkms_metabolic/Pistachio_ringbreaker/Reaxys/Reaxys_biocatalysis |
Top-N result to add to graph | 6 |
Feasible Synthetic Routes
Disclaimer and Information on In-Vitro Research Products
Please be aware that all articles and product information presented on BenchChem are intended solely for informational purposes. The products available for purchase on BenchChem are specifically designed for in-vitro studies, which are conducted outside of living organisms. In-vitro studies, derived from the Latin term "in glass," involve experiments performed in controlled laboratory settings using cells or tissues. It is important to note that these products are not categorized as medicines or drugs, and they have not received approval from the FDA for the prevention, treatment, or cure of any medical condition, ailment, or disease. We must emphasize that any form of bodily introduction of these products into humans or animals is strictly prohibited by law. It is essential to adhere to these guidelines to ensure compliance with legal and ethical standards in research and experimentation.