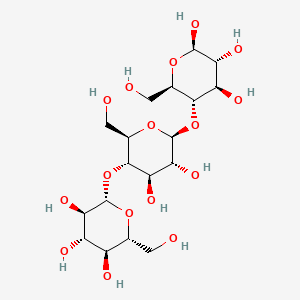
Cellulase
Overview
Description
Cellulase is a group of enzymes that catalyze the breakdown of cellulose into glucose and other oligosaccharides. These enzymes are primarily produced by fungi, bacteria, and protozoans. This compound plays a crucial role in the decomposition of cellulose, a major component of plant cell walls, making it available for various biological and industrial processes .
Mechanism of Action
Target of Action
Cellulase, also known as beta-cellotriose, primarily targets cellulose, the most abundant polysaccharide present on earth . This compound is a complex group of enzymes secreted by a broad range of microorganisms including fungi, bacteria, and actinomycetes . The enzyme targets the β-1,4-linkages in the polymeric structure of cellulose . The primary targets of this compound are the endoglucanases, exoglucanases, and β-glucosidases .
Mode of Action
This compound interacts with its targets through a process known as cellulolysis, the decomposition of cellulose and some related polysaccharides . The specific reaction involved is the hydrolysis of the 1,4-β-D-glycosidic linkages in cellulose . The exoglucanase acts on the reducing ends of the cellulose chain and releases cellobiose as the end product . Endoglucanase randomly attacks the internal o-glycosidic bonds, resulting in glucan chains of different lengths . The β-glucosidases act specifically on the β-cellobiose disaccharides and produce glucose .
Biochemical Pathways
The biochemical pathway of this compound involves the synergistic action of three types of enzymatic activities: endoglucanases or 1, 4-β-D-glucan 4-glucanohydrolases, exoglucanases, and β-glucosidases or β-D-glucoside glucohydrolases . This results in the release of D-glucose units from soluble cellodextrins and a variety of glycosides . The glucose units can then be fermented to bioethanol .
Pharmacokinetics
The pharmacokinetics of this compound involve the understanding of accurate this compound catalytic activities . The process of this compound biocatalysis is considered a heterogeneous reaction where crystallinity has been shown to be a key parameter . The processivity, synergism, and mechanistic paradigm for cellulose depolymerizations are critical factors that influence the bioavailability of the products of this compound action .
Result of Action
The result of this compound action is the breakdown of the cellulose molecule into monosaccharides (“simple sugars”) such as β-glucose, or shorter polysaccharides and oligosaccharides .
Action Environment
In the natural environment, synergistic interactions among cellulolytic microorganisms play an important role in the hydrolysis of lignocellulosic polymer materials . The efficiency of this process is determined by the combined action of three major enzymes: exoglucanases, endoglucanases, and β-glucosidase . The action pathway of cellulases mainly depends on the distance of the catalytic sites . About 90-95% of all bacterial this compound activity is observed under aerobic conditions by aerobic bacteria .
Biochemical Analysis
Biochemical Properties
Cellulase catalyzes the endohydrolysis of 1,4-beta-D-glucosidic linkages in cellulose, lichenin, and cereal beta-D-glucans . It breaks down the cellulose molecule into monosaccharides such as beta-glucose, or shorter polysaccharides and oligosaccharides . The specific reaction involved is the hydrolysis of the 1,4-beta-D-glycosidic linkages in cellulose, hemicellulose, lichenin, and cereal beta-D-glucans .
Cellular Effects
This compound has been found to have antioxidant properties, which may help protect against damaging free radicals . Free radicals steal electrons from healthy cells, resulting in damage to the cellular structure. Prolonged cellular damage can accelerate aging and increase one’s risk for various diseases .
Molecular Mechanism
This compound decomposes cellulose through a process called cellulolysis. This involves the endohydrolysis of 1,4-beta-D-glucosidic linkages in cellulose, lichenin, and cereal beta-D-glucans . The specific reaction involved is the hydrolysis of the 1,4-beta-D-glycosidic linkages in cellulose, hemicellulose, lichenin, and cereal beta-D-glucans .
Temporal Effects in Laboratory Settings
In laboratory settings, the effects of this compound can change over time. For instance, the study conducted by Pang et al. found that the peak activity of this compound reached 14.04±0.42 U/ml utilizing statistical optimization using Response Surface Methodology (RSM) after 72 hours of fermentation .
Dosage Effects in Animal Models
The effects of this compound can vary with different dosages in animal models. For instance, dairy cows fed with forage treated with a this compound enzyme preparation ate more feed and produced 5-25% more milk .
Metabolic Pathways
This compound plays a key role in the metabolic pathway of cellulose degradation. It catalyzes the endohydrolysis of 1,4-beta-D-glucosidic linkages in cellulose, lichenin, and cereal beta-D-glucans .
Transport and Distribution
This compound goes through secretory pathways to reach the extracellular space, where enzymatic reactions take place . Secretory pathways in various cells have been the focus of many research fields .
Subcellular Localization
This compound is located in the endoplasmic reticulum, Golgi, vacuoles, and cell membrane/wall, but not the septum . It is secreted abundantly into the culture medium .
Preparation Methods
Synthetic Routes and Reaction Conditions
Cellulase enzymes are typically produced through microbial fermentation. The primary sources include fungi such as Trichoderma reesei and bacteria like Bacillus subtilis. The production process involves cultivating these microorganisms in a nutrient-rich medium under controlled conditions. The enzymes are then extracted and purified using techniques such as filtration, centrifugation, and chromatography .
Industrial Production Methods
Industrial production of this compound involves large-scale fermentation processes. Submerged fermentation and solid-state fermentation are the two main methods used. Submerged fermentation is carried out in liquid media, while solid-state fermentation uses solid substrates like agricultural waste. The choice of method depends on the desired enzyme yield and application .
Chemical Reactions Analysis
Types of Reactions
Cellulase primarily catalyzes the hydrolysis of β-1,4-glycosidic bonds in cellulose. This reaction involves the addition of water molecules to break the bonds, resulting in the formation of glucose and other oligosaccharides .
Common Reagents and Conditions
The hydrolysis reaction catalyzed by this compound typically occurs under mild conditions, such as a pH range of 4.5 to 5.5 and temperatures between 40°C to 50°C. Common reagents include buffer solutions like sodium acetate to maintain the optimal pH .
Major Products
The primary products of this compound-catalyzed reactions are glucose, cellobiose, and other oligosaccharides. These products are essential for various biological and industrial processes, including biofuel production and food processing .
Scientific Research Applications
Cellulase has a wide range of applications in scientific research and industry:
Biofuel Production: This compound is used to convert lignocellulosic biomass into fermentable sugars, which are then used to produce bioethanol
Food and Feed Industry: It is used to improve the digestibility of animal feed and to clarify fruit juices
Textile Industry: This compound is employed in the biopolishing of fabrics and the production of stone-washed denim
Paper and Pulp Industry: It is used in the deinking of recycled paper and the enhancement of paper quality
Medical and Pharmaceutical Applications: This compound is used in the extraction of carotenoids and in the production of protoplasts for genetic engineering.
Comparison with Similar Compounds
Cellulase is part of a broader group of enzymes known as glycoside hydrolases. Similar enzymes include:
Hemithis compound: Catalyzes the breakdown of hemicellulose, another major component of plant cell walls
Pectinase: Breaks down pectin, a polysaccharide found in plant cell walls.
This compound is unique in its ability to specifically target cellulose, making it indispensable for processes that require the breakdown of this complex polysaccharide .
Properties
IUPAC Name |
(2S,3R,4S,5S,6R)-2-[(2R,3S,4R,5R,6S)-4,5-dihydroxy-2-(hydroxymethyl)-6-[(2R,3S,4R,5R,6R)-4,5,6-trihydroxy-2-(hydroxymethyl)oxan-3-yl]oxyoxan-3-yl]oxy-6-(hydroxymethyl)oxane-3,4,5-triol | |
---|---|---|
Details | Computed by Lexichem TK 2.7.0 (PubChem release 2021.05.07) | |
Source | PubChem | |
URL | https://pubchem.ncbi.nlm.nih.gov | |
Description | Data deposited in or computed by PubChem | |
InChI |
InChI=1S/C18H32O16/c19-1-4-7(22)8(23)12(27)17(31-4)34-15-6(3-21)32-18(13(28)10(15)25)33-14-5(2-20)30-16(29)11(26)9(14)24/h4-29H,1-3H2/t4-,5-,6-,7-,8+,9-,10-,11-,12-,13-,14-,15-,16-,17+,18+/m1/s1 | |
Details | Computed by InChI 1.0.6 (PubChem release 2021.05.07) | |
Source | PubChem | |
URL | https://pubchem.ncbi.nlm.nih.gov | |
Description | Data deposited in or computed by PubChem | |
InChI Key |
FYGDTMLNYKFZSV-CSHPIKHBSA-N | |
Details | Computed by InChI 1.0.6 (PubChem release 2021.05.07) | |
Source | PubChem | |
URL | https://pubchem.ncbi.nlm.nih.gov | |
Description | Data deposited in or computed by PubChem | |
Canonical SMILES |
C(C1C(C(C(C(O1)OC2C(OC(C(C2O)O)OC3C(OC(C(C3O)O)O)CO)CO)O)O)O)O | |
Details | Computed by OEChem 2.3.0 (PubChem release 2021.05.07) | |
Source | PubChem | |
URL | https://pubchem.ncbi.nlm.nih.gov | |
Description | Data deposited in or computed by PubChem | |
Isomeric SMILES |
C([C@@H]1[C@H]([C@@H]([C@H]([C@@H](O1)O[C@@H]2[C@H](O[C@H]([C@@H]([C@H]2O)O)O[C@@H]3[C@H](O[C@H]([C@@H]([C@H]3O)O)O)CO)CO)O)O)O)O | |
Details | Computed by OEChem 2.3.0 (PubChem release 2021.05.07) | |
Source | PubChem | |
URL | https://pubchem.ncbi.nlm.nih.gov | |
Description | Data deposited in or computed by PubChem | |
Molecular Formula |
C18H32O16 | |
Details | Computed by PubChem 2.1 (PubChem release 2021.05.07) | |
Source | PubChem | |
URL | https://pubchem.ncbi.nlm.nih.gov | |
Description | Data deposited in or computed by PubChem | |
Molecular Weight |
504.4 g/mol | |
Details | Computed by PubChem 2.1 (PubChem release 2021.05.07) | |
Source | PubChem | |
URL | https://pubchem.ncbi.nlm.nih.gov | |
Description | Data deposited in or computed by PubChem | |
Physical Description |
Solid; [IUCLID] | |
Record name | Cellulase | |
Source | Haz-Map, Information on Hazardous Chemicals and Occupational Diseases | |
URL | https://haz-map.com/Agents/12798 | |
Description | Haz-Map® is an occupational health database designed for health and safety professionals and for consumers seeking information about the adverse effects of workplace exposures to chemical and biological agents. | |
Explanation | Copyright (c) 2022 Haz-Map(R). All rights reserved. Unless otherwise indicated, all materials from Haz-Map are copyrighted by Haz-Map(R). No part of these materials, either text or image may be used for any purpose other than for personal use. Therefore, reproduction, modification, storage in a retrieval system or retransmission, in any form or by any means, electronic, mechanical or otherwise, for reasons other than personal use, is strictly prohibited without prior written permission. | |
CAS No. |
9012-54-8, 61788-77-0 | |
Record name | Cellulase | |
Source | EPA Chemicals under the TSCA | |
URL | https://www.epa.gov/chemicals-under-tsca | |
Description | EPA Chemicals under the Toxic Substances Control Act (TSCA) collection contains information on chemicals and their regulations under TSCA, including non-confidential content from the TSCA Chemical Substance Inventory and Chemical Data Reporting. | |
Record name | Cellulase | |
Source | European Chemicals Agency (ECHA) | |
URL | https://echa.europa.eu/substance-information/-/substanceinfo/100.029.746 | |
Description | The European Chemicals Agency (ECHA) is an agency of the European Union which is the driving force among regulatory authorities in implementing the EU's groundbreaking chemicals legislation for the benefit of human health and the environment as well as for innovation and competitiveness. | |
Explanation | Use of the information, documents and data from the ECHA website is subject to the terms and conditions of this Legal Notice, and subject to other binding limitations provided for under applicable law, the information, documents and data made available on the ECHA website may be reproduced, distributed and/or used, totally or in part, for non-commercial purposes provided that ECHA is acknowledged as the source: "Source: European Chemicals Agency, http://echa.europa.eu/". Such acknowledgement must be included in each copy of the material. ECHA permits and encourages organisations and individuals to create links to the ECHA website under the following cumulative conditions: Links can only be made to webpages that provide a link to the Legal Notice page. | |
Record name | Rayon | |
Source | European Chemicals Agency (ECHA) | |
URL | https://echa.europa.eu/information-on-chemicals | |
Description | The European Chemicals Agency (ECHA) is an agency of the European Union which is the driving force among regulatory authorities in implementing the EU's groundbreaking chemicals legislation for the benefit of human health and the environment as well as for innovation and competitiveness. | |
Explanation | Use of the information, documents and data from the ECHA website is subject to the terms and conditions of this Legal Notice, and subject to other binding limitations provided for under applicable law, the information, documents and data made available on the ECHA website may be reproduced, distributed and/or used, totally or in part, for non-commercial purposes provided that ECHA is acknowledged as the source: "Source: European Chemicals Agency, http://echa.europa.eu/". Such acknowledgement must be included in each copy of the material. ECHA permits and encourages organisations and individuals to create links to the ECHA website under the following cumulative conditions: Links can only be made to webpages that provide a link to the Legal Notice page. | |
Disclaimer and Information on In-Vitro Research Products
Please be aware that all articles and product information presented on BenchChem are intended solely for informational purposes. The products available for purchase on BenchChem are specifically designed for in-vitro studies, which are conducted outside of living organisms. In-vitro studies, derived from the Latin term "in glass," involve experiments performed in controlled laboratory settings using cells or tissues. It is important to note that these products are not categorized as medicines or drugs, and they have not received approval from the FDA for the prevention, treatment, or cure of any medical condition, ailment, or disease. We must emphasize that any form of bodily introduction of these products into humans or animals is strictly prohibited by law. It is essential to adhere to these guidelines to ensure compliance with legal and ethical standards in research and experimentation.