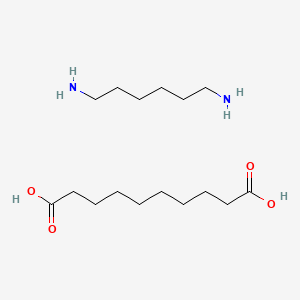
Hexamethylenediamine sebacate
Overview
Description
Hexamethylenediamine sebacate is a chemical compound formed by the reaction of hexamethylenediamine and sebacic acid. It is commonly used in the production of nylon and other polyamides due to its ability to form strong, durable polymers. The compound is known for its high melting point and excellent mechanical properties, making it a valuable material in various industrial applications.
Preparation Methods
Synthetic Routes and Reaction Conditions: Hexamethylenediamine sebacate is synthesized through a polycondensation reaction between hexamethylenediamine and sebacic acid. The reaction typically occurs in a solvent-free environment at elevated temperatures, often around 200-250°C, to facilitate the removal of water and drive the reaction to completion. The process may involve the use of catalysts to enhance the reaction rate and improve yield.
Industrial Production Methods: In industrial settings, the production of this compound involves large-scale reactors where the reactants are continuously fed, and the product is continuously removed. The process is optimized to ensure high purity and yield, often incorporating steps such as distillation and crystallization to purify the final product. The use of advanced monitoring and control systems ensures consistent quality and efficiency in production.
Chemical Reactions Analysis
Types of Reactions: Hexamethylenediamine sebacate undergoes various chemical reactions, including:
Oxidation: The compound can be oxidized under specific conditions to form corresponding oxides.
Reduction: Reduction reactions can convert this compound into its reduced forms, often using hydrogen gas and metal catalysts.
Substitution: The amine groups in this compound can participate in nucleophilic substitution reactions, where other functional groups replace the amine groups.
Common Reagents and Conditions:
Oxidation: Common oxidizing agents include potassium permanganate and hydrogen peroxide, typically used under acidic or basic conditions.
Reduction: Hydrogen gas in the presence of catalysts such as palladium or nickel is commonly used for reduction reactions.
Substitution: Nucleophilic substitution reactions often involve reagents like alkyl halides or acyl chlorides under controlled temperature and pressure conditions.
Major Products:
Oxidation: Oxidized derivatives of this compound.
Reduction: Reduced forms of the compound, such as hexamethylenediamine and sebacic acid derivatives.
Substitution: Substituted products with various functional groups replacing the amine groups.
Scientific Research Applications
Hexamethylenediamine sebacate has a wide range of applications in scientific research, including:
Chemistry: Used as a monomer in the synthesis of polyamides and other polymers. It is also a key intermediate in the production of various chemical compounds.
Biology: Employed in the development of biomaterials and tissue engineering scaffolds due to its biocompatibility and mechanical strength.
Medicine: Investigated for its potential use in drug delivery systems and medical devices, leveraging its stability and compatibility with biological systems.
Industry: Widely used in the production of nylon 66, a high-performance polymer with applications in textiles, automotive parts, and consumer goods.
Mechanism of Action
The mechanism of action of hexamethylenediamine sebacate involves its ability to form strong hydrogen bonds and covalent bonds with other molecules. The amine groups in this compound can interact with various molecular targets, facilitating the formation of stable polymers and other compounds. The pathways involved include polycondensation reactions, where the compound reacts with dicarboxylic acids to form long-chain polymers with high mechanical strength and thermal stability.
Comparison with Similar Compounds
Hexamethylenediamine sebacate can be compared with other similar compounds such as:
Adipic acid and hexamethylenediamine: Used in the production of nylon 66, similar to this compound but with different mechanical properties.
1,6-Diaminohexane: Another diamine used in polymer synthesis, offering different reactivity and properties compared to this compound.
Sebacoyl chloride: A derivative of sebacic acid used in the production of polyamides and other polymers, providing different chemical reactivity and applications.
This compound stands out due to its unique combination of mechanical strength, thermal stability, and versatility in various applications, making it a valuable compound in both research and industrial settings.
Properties
IUPAC Name |
decanedioic acid;hexane-1,6-diamine | |
---|---|---|
Details | Computed by Lexichem TK 2.7.0 (PubChem release 2021.05.07) | |
Source | PubChem | |
URL | https://pubchem.ncbi.nlm.nih.gov | |
Description | Data deposited in or computed by PubChem | |
InChI |
InChI=1S/C10H18O4.C6H16N2/c11-9(12)7-5-3-1-2-4-6-8-10(13)14;7-5-3-1-2-4-6-8/h1-8H2,(H,11,12)(H,13,14);1-8H2 | |
Details | Computed by InChI 1.0.6 (PubChem release 2021.05.07) | |
Source | PubChem | |
URL | https://pubchem.ncbi.nlm.nih.gov | |
Description | Data deposited in or computed by PubChem | |
InChI Key |
YWJUZWOHLHBWQY-UHFFFAOYSA-N | |
Details | Computed by InChI 1.0.6 (PubChem release 2021.05.07) | |
Source | PubChem | |
URL | https://pubchem.ncbi.nlm.nih.gov | |
Description | Data deposited in or computed by PubChem | |
Canonical SMILES |
C(CCCCC(=O)O)CCCC(=O)O.C(CCCN)CCN | |
Details | Computed by OEChem 2.3.0 (PubChem release 2021.05.07) | |
Source | PubChem | |
URL | https://pubchem.ncbi.nlm.nih.gov | |
Description | Data deposited in or computed by PubChem | |
Molecular Formula |
C16H34N2O4 | |
Details | Computed by PubChem 2.1 (PubChem release 2021.05.07) | |
Source | PubChem | |
URL | https://pubchem.ncbi.nlm.nih.gov | |
Description | Data deposited in or computed by PubChem | |
Related CAS |
52349-39-0, 6422-99-7, 9011-52-3 | |
Details | Compound: Decanedioic acid, polymer with 1,6-hexanediamine | |
Record name | Decanedioic acid, compd. with 1,6-hexanediamine (1:1), homopolymer | |
Source | CAS Common Chemistry | |
URL | https://commonchemistry.cas.org/detail?cas_rn=52349-39-0 | |
Description | CAS Common Chemistry is an open community resource for accessing chemical information. Nearly 500,000 chemical substances from CAS REGISTRY cover areas of community interest, including common and frequently regulated chemicals, and those relevant to high school and undergraduate chemistry classes. This chemical information, curated by our expert scientists, is provided in alignment with our mission as a division of the American Chemical Society. | |
Explanation | The data from CAS Common Chemistry is provided under a CC-BY-NC 4.0 license, unless otherwise stated. | |
Details | Compound: Decanedioic acid, polymer with 1,6-hexanediamine | |
Record name | Decanedioic acid, compd. with 1,6-hexanediamine (1:1) | |
Source | CAS Common Chemistry | |
URL | https://commonchemistry.cas.org/detail?cas_rn=6422-99-7 | |
Description | CAS Common Chemistry is an open community resource for accessing chemical information. Nearly 500,000 chemical substances from CAS REGISTRY cover areas of community interest, including common and frequently regulated chemicals, and those relevant to high school and undergraduate chemistry classes. This chemical information, curated by our expert scientists, is provided in alignment with our mission as a division of the American Chemical Society. | |
Explanation | The data from CAS Common Chemistry is provided under a CC-BY-NC 4.0 license, unless otherwise stated. | |
Details | Compound: Decanedioic acid, polymer with 1,6-hexanediamine | |
Record name | Decanedioic acid, polymer with 1,6-hexanediamine | |
Source | CAS Common Chemistry | |
URL | https://commonchemistry.cas.org/detail?cas_rn=9011-52-3 | |
Description | CAS Common Chemistry is an open community resource for accessing chemical information. Nearly 500,000 chemical substances from CAS REGISTRY cover areas of community interest, including common and frequently regulated chemicals, and those relevant to high school and undergraduate chemistry classes. This chemical information, curated by our expert scientists, is provided in alignment with our mission as a division of the American Chemical Society. | |
Explanation | The data from CAS Common Chemistry is provided under a CC-BY-NC 4.0 license, unless otherwise stated. | |
DSSTOX Substance ID |
DTXSID2027626 | |
Record name | Decanedioic acid, compd. with 1,6-hexanediamine (1:1) | |
Source | EPA DSSTox | |
URL | https://comptox.epa.gov/dashboard/DTXSID2027626 | |
Description | DSSTox provides a high quality public chemistry resource for supporting improved predictive toxicology. | |
Molecular Weight |
318.45 g/mol | |
Details | Computed by PubChem 2.1 (PubChem release 2021.05.07) | |
Source | PubChem | |
URL | https://pubchem.ncbi.nlm.nih.gov | |
Description | Data deposited in or computed by PubChem | |
CAS No. |
6422-99-7, 9011-52-3 | |
Record name | Hexamethylenediamine sebacate | |
Source | ChemIDplus | |
URL | https://pubchem.ncbi.nlm.nih.gov/substance/?source=chemidplus&sourceid=0006422997 | |
Description | ChemIDplus is a free, web search system that provides access to the structure and nomenclature authority files used for the identification of chemical substances cited in National Library of Medicine (NLM) databases, including the TOXNET system. | |
Record name | Decanedioic acid, polymer with 1,6-hexanediamine | |
Source | ChemIDplus | |
URL | https://pubchem.ncbi.nlm.nih.gov/substance/?source=chemidplus&sourceid=0009011523 | |
Description | ChemIDplus is a free, web search system that provides access to the structure and nomenclature authority files used for the identification of chemical substances cited in National Library of Medicine (NLM) databases, including the TOXNET system. | |
Record name | Decanedioic acid, compd. with 1,6-hexanediamine (1:1) | |
Source | EPA Chemicals under the TSCA | |
URL | https://www.epa.gov/chemicals-under-tsca | |
Description | EPA Chemicals under the Toxic Substances Control Act (TSCA) collection contains information on chemicals and their regulations under TSCA, including non-confidential content from the TSCA Chemical Substance Inventory and Chemical Data Reporting. | |
Record name | Decanedioic acid, polymer with 1,6-hexanediamine | |
Source | EPA Chemicals under the TSCA | |
URL | https://www.epa.gov/chemicals-under-tsca | |
Description | EPA Chemicals under the Toxic Substances Control Act (TSCA) collection contains information on chemicals and their regulations under TSCA, including non-confidential content from the TSCA Chemical Substance Inventory and Chemical Data Reporting. | |
Record name | Decanedioic acid, compd. with 1,6-hexanediamine (1:1) | |
Source | EPA DSSTox | |
URL | https://comptox.epa.gov/dashboard/DTXSID2027626 | |
Description | DSSTox provides a high quality public chemistry resource for supporting improved predictive toxicology. | |
Record name | Sebacic acid, compound with hexane-1,6-diamine (1:1) | |
Source | European Chemicals Agency (ECHA) | |
URL | https://echa.europa.eu/substance-information/-/substanceinfo/100.026.525 | |
Description | The European Chemicals Agency (ECHA) is an agency of the European Union which is the driving force among regulatory authorities in implementing the EU's groundbreaking chemicals legislation for the benefit of human health and the environment as well as for innovation and competitiveness. | |
Explanation | Use of the information, documents and data from the ECHA website is subject to the terms and conditions of this Legal Notice, and subject to other binding limitations provided for under applicable law, the information, documents and data made available on the ECHA website may be reproduced, distributed and/or used, totally or in part, for non-commercial purposes provided that ECHA is acknowledged as the source: "Source: European Chemicals Agency, http://echa.europa.eu/". Such acknowledgement must be included in each copy of the material. ECHA permits and encourages organisations and individuals to create links to the ECHA website under the following cumulative conditions: Links can only be made to webpages that provide a link to the Legal Notice page. | |
Record name | HEXAMETHYLENEDIAMINE SEBACATE | |
Source | FDA Global Substance Registration System (GSRS) | |
URL | https://gsrs.ncats.nih.gov/ginas/app/beta/substances/62G464KX7Q | |
Description | The FDA Global Substance Registration System (GSRS) enables the efficient and accurate exchange of information on what substances are in regulated products. Instead of relying on names, which vary across regulatory domains, countries, and regions, the GSRS knowledge base makes it possible for substances to be defined by standardized, scientific descriptions. | |
Explanation | Unless otherwise noted, the contents of the FDA website (www.fda.gov), both text and graphics, are not copyrighted. They are in the public domain and may be republished, reprinted and otherwise used freely by anyone without the need to obtain permission from FDA. Credit to the U.S. Food and Drug Administration as the source is appreciated but not required. | |
Retrosynthesis Analysis
AI-Powered Synthesis Planning: Our tool employs the Template_relevance Pistachio, Template_relevance Bkms_metabolic, Template_relevance Pistachio_ringbreaker, Template_relevance Reaxys, Template_relevance Reaxys_biocatalysis model, leveraging a vast database of chemical reactions to predict feasible synthetic routes.
One-Step Synthesis Focus: Specifically designed for one-step synthesis, it provides concise and direct routes for your target compounds, streamlining the synthesis process.
Accurate Predictions: Utilizing the extensive PISTACHIO, BKMS_METABOLIC, PISTACHIO_RINGBREAKER, REAXYS, REAXYS_BIOCATALYSIS database, our tool offers high-accuracy predictions, reflecting the latest in chemical research and data.
Strategy Settings
Precursor scoring | Relevance Heuristic |
---|---|
Min. plausibility | 0.01 |
Model | Template_relevance |
Template Set | Pistachio/Bkms_metabolic/Pistachio_ringbreaker/Reaxys/Reaxys_biocatalysis |
Top-N result to add to graph | 6 |
Feasible Synthetic Routes
Disclaimer and Information on In-Vitro Research Products
Please be aware that all articles and product information presented on BenchChem are intended solely for informational purposes. The products available for purchase on BenchChem are specifically designed for in-vitro studies, which are conducted outside of living organisms. In-vitro studies, derived from the Latin term "in glass," involve experiments performed in controlled laboratory settings using cells or tissues. It is important to note that these products are not categorized as medicines or drugs, and they have not received approval from the FDA for the prevention, treatment, or cure of any medical condition, ailment, or disease. We must emphasize that any form of bodily introduction of these products into humans or animals is strictly prohibited by law. It is essential to adhere to these guidelines to ensure compliance with legal and ethical standards in research and experimentation.