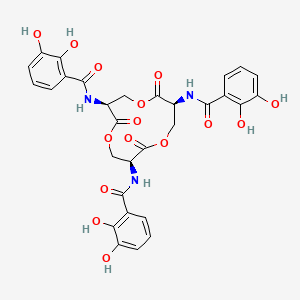
Enterobactin
Overview
Description
It is recognized for its exceptional ability to chelate ferric ions (Fe³⁺) with an affinity constant (K) of approximately 10⁵² M⁻¹, making it one of the strongest known siderophores . This compound plays a crucial role in iron acquisition for microbial systems, especially in environments where iron availability is extremely low.
Mechanism of Action
- The (pro)renin receptor is involved in renin’s enzymatic activity, specifically hydrolyzing angiotensinogen to angiotensin I .
- Downstream effects include arterial vasoconstriction , which helps restore perfusion pressure in the kidneys .
- It is secreted via both a constitutive pathway (for prorenin) and a regulated pathway (for mature renin) .
Target of Action
Mode of Action
Biochemical Pathways
Pharmacokinetics
Result of Action
Biochemical Analysis
Biochemical Properties
Rennin plays a crucial role in biochemical reactions, particularly in the digestion of proteins. It interacts with caseinogen, a protein found in milk, and transforms it into insoluble casein, thereby curdling the milk . This interaction is a key step in the digestion process of young ruminants.
Cellular Effects
The effects of rennin at the cellular level are primarily observed in the cells lining the stomach of young ruminants. Here, rennin aids in the digestion process by curdling milk, which slows down the rate at which the milk leaves the stomach, thereby allowing more time for digestion and absorption of nutrients .
Molecular Mechanism
Rennin exerts its effects at the molecular level through its enzymatic activity. It binds to the protein caseinogen and catalyzes its transformation into insoluble casein . This enzymatic reaction is specific and does not involve the inhibition or activation of other enzymes.
Temporal Effects in Laboratory Settings
In laboratory settings, the effects of rennin can be observed over time as the milk curdles. The stability of rennin and its resistance to degradation allow it to continue acting on caseinogen for an extended period, leading to the formation of larger curds .
Dosage Effects in Animal Models
In animal models, particularly young ruminants, the effects of rennin are dose-dependent. A sufficient amount of rennin is necessary to curdle the milk consumed by the animal effectively. Excessive amounts of rennin do not typically lead to adverse effects, as the enzyme is specific to its substrate, caseinogen .
Metabolic Pathways
Rennin is involved in the metabolic pathway of protein digestion in ruminants. It interacts with the protein caseinogen, transforming it into insoluble casein and thus initiating the process of protein digestion .
Transport and Distribution
Rennin is produced in the cells of the gastric mucosa in the fourth stomach of young ruminants and is secreted into the stomach where it acts on the milk consumed by the animal .
Subcellular Localization
Rennin is localized in the secretory vesicles of the gastric mucosal cells. Upon secretion, it enters the stomach where it acts on the caseinogen present in the milk .
Preparation Methods
Synthetic Routes and Reaction Conditions: Enterobactin is synthesized through a series of enzymatic reactions starting from chorismic acid, an aromatic amino acid precursor . The biosynthesis involves the conversion of chorismic acid to 2,3-dihydroxybenzoic acid (DHB) by the enzymes EntA, EntB, and EntC. Subsequently, DHB is linked to L-serine via amide bonds catalyzed by EntD, EntE, EntF, and EntB. The final step involves the cyclization of three DHB-Ser molecules to form this compound .
Industrial Production Methods: While industrial production methods for this compound are not extensively documented, the biosynthetic pathway in bacteria can be harnessed for large-scale production. Genetic engineering techniques can be employed to overexpress the relevant biosynthetic genes in suitable microbial hosts, optimizing conditions for maximum yield.
Chemical Reactions Analysis
Types of Reactions: Enterobactin undergoes various chemical reactions, including:
Oxidation: this compound can act as an oxidative stress protector by scavenging reactive oxygen species.
Reduction: The iron-bound form of this compound can be reduced to release iron within the bacterial cell.
Substitution: this compound can form conjugates with other molecules, such as antibiotics, to enhance their uptake and efficacy.
Common Reagents and Conditions:
Oxidation: Hydrogen peroxide (H₂O₂) is commonly used to induce oxidative stress in studies involving this compound.
Reduction: Reducing agents like ascorbic acid can facilitate the reduction of iron-bound this compound.
Substitution: Polyethylene glycol (PEG) linkers are used to attach antibiotics to this compound.
Major Products Formed:
Oxidation: Hydrolyzed this compound with exposed hydroxyl groups.
Reduction: Free iron and reduced this compound.
Substitution: this compound-antibiotic conjugates.
Scientific Research Applications
Enterobactin has diverse applications in scientific research:
Comparison with Similar Compounds
Enterobactin is unique among siderophores due to its high affinity for ferric ions and its cyclic trimer structure composed of 2,3-dihydroxy-N-benzoylserine . Similar compounds include:
Ferrioxamine B: A trihydroxamate siderophore produced by Gram-positive bacteria.
Azotochelin: A catecholate siderophore produced by Azotobacter vinelandii.
Myxochelin A: A catecholate siderophore produced by the myxobacterium Angiococcus disciformis.
These compounds differ in their structural features and the types of bacteria that produce them, highlighting the diversity of siderophore-mediated iron acquisition strategies in different microbial species.
Properties
IUPAC Name |
N-[7,11-bis[(2,3-dihydroxybenzoyl)amino]-2,6,10-trioxo-1,5,9-trioxacyclododec-3-yl]-2,3-dihydroxybenzamide | |
---|---|---|
Details | Computed by Lexichem TK 2.7.0 (PubChem release 2021.05.07) | |
Source | PubChem | |
URL | https://pubchem.ncbi.nlm.nih.gov | |
Description | Data deposited in or computed by PubChem | |
InChI |
InChI=1S/C30H27N3O15/c34-19-7-1-4-13(22(19)37)25(40)31-16-10-46-29(44)18(33-27(42)15-6-3-9-21(36)24(15)39)12-48-30(45)17(11-47-28(16)43)32-26(41)14-5-2-8-20(35)23(14)38/h1-9,16-18,34-39H,10-12H2,(H,31,40)(H,32,41)(H,33,42) | |
Details | Computed by InChI 1.0.6 (PubChem release 2021.05.07) | |
Source | PubChem | |
URL | https://pubchem.ncbi.nlm.nih.gov | |
Description | Data deposited in or computed by PubChem | |
InChI Key |
SERBHKJMVBATSJ-UHFFFAOYSA-N | |
Details | Computed by InChI 1.0.6 (PubChem release 2021.05.07) | |
Source | PubChem | |
URL | https://pubchem.ncbi.nlm.nih.gov | |
Description | Data deposited in or computed by PubChem | |
Canonical SMILES |
C1C(C(=O)OCC(C(=O)OCC(C(=O)O1)NC(=O)C2=C(C(=CC=C2)O)O)NC(=O)C3=C(C(=CC=C3)O)O)NC(=O)C4=C(C(=CC=C4)O)O | |
Details | Computed by OEChem 2.3.0 (PubChem release 2021.05.07) | |
Source | PubChem | |
URL | https://pubchem.ncbi.nlm.nih.gov | |
Description | Data deposited in or computed by PubChem | |
Molecular Formula |
C30H27N3O15 | |
Details | Computed by PubChem 2.1 (PubChem release 2021.05.07) | |
Source | PubChem | |
URL | https://pubchem.ncbi.nlm.nih.gov | |
Description | Data deposited in or computed by PubChem | |
Molecular Weight |
669.5 g/mol | |
Details | Computed by PubChem 2.1 (PubChem release 2021.05.07) | |
Source | PubChem | |
URL | https://pubchem.ncbi.nlm.nih.gov | |
Description | Data deposited in or computed by PubChem | |
Retrosynthesis Analysis
AI-Powered Synthesis Planning: Our tool employs the Template_relevance Pistachio, Template_relevance Bkms_metabolic, Template_relevance Pistachio_ringbreaker, Template_relevance Reaxys, Template_relevance Reaxys_biocatalysis model, leveraging a vast database of chemical reactions to predict feasible synthetic routes.
One-Step Synthesis Focus: Specifically designed for one-step synthesis, it provides concise and direct routes for your target compounds, streamlining the synthesis process.
Accurate Predictions: Utilizing the extensive PISTACHIO, BKMS_METABOLIC, PISTACHIO_RINGBREAKER, REAXYS, REAXYS_BIOCATALYSIS database, our tool offers high-accuracy predictions, reflecting the latest in chemical research and data.
Strategy Settings
Precursor scoring | Relevance Heuristic |
---|---|
Min. plausibility | 0.01 |
Model | Template_relevance |
Template Set | Pistachio/Bkms_metabolic/Pistachio_ringbreaker/Reaxys/Reaxys_biocatalysis |
Top-N result to add to graph | 6 |
Feasible Synthetic Routes
Q1: What is Rennin and what is its primary function?
A: Rennin, also known as chymosin (EC 3.4.4.3), is an aspartic protease primarily known for its role in cheesemaking. It is synthesized by gastric cells in the stomachs of young mammals, including calves, where it plays a crucial role in milk digestion. [, ]
Q2: How does Rennin interact with its target, and what are the downstream effects of this interaction?
A: Rennin exhibits high specificity for cleaving a single peptide bond (Phe105-Met106) in kappa-casein, a protein crucial for stabilizing milk micelles. [, , ] This cleavage results in the formation of para-kappa-casein and glycomacropeptide. [, , , , ] Para-kappa-casein, lacking the stabilizing glycomacropeptide, aggregates with other casein molecules in the presence of calcium ions, leading to milk coagulation and the formation of curd. [, , , ] This specific cleavage is crucial for the texture and flavor development in cheese. [, ]
Q3: What is known about the structural characterization of Rennin?
A: While the exact molecular formula and weight can vary slightly depending on the source and purification method, Rennin is a single-chain protein composed of approximately 323 amino acids with a molecular weight around 35 kDa. [, , ] Detailed structural information, including spectroscopic data, can be found in research on its crystallography. [] Researchers have successfully crystallized calf rennin, revealing the presence of a stable orthorhombic form (Rennin I). []
Q4: How does pH affect Rennin's activity and stability?
A: Rennin exhibits optimal activity in slightly acidic environments. [, , , , ] Its stability is highly influenced by pH, with the presence of other proteases like pepsin significantly impacting its degradation at low pH values. [, ] Research suggests that Rennin is most stable in blended solutions between pH 5.5 and 6.5. []
Q5: What is the impact of temperature on Rennin's performance?
A: Temperature plays a significant role in Rennin's activity, with an optimal temperature range for milk clotting typically observed. [, ] Studies investigating milk coagulation using the Formagraph demonstrate the impact of varying temperatures on both coagulation time and curd firmness. []
Q6: What are the primary applications of Rennin?
A: The most prominent application of Rennin is in the cheesemaking industry, where it serves as a crucial coagulant for milk. [, , ] It's ability to specifically cleave kappa-casein makes it highly valuable for producing various cheese varieties. [, , ]
Q7: Are there alternatives to animal-derived Rennin?
A: Yes, the cheesemaking industry utilizes alternative milk-clotting enzymes derived from microbial sources, such as Mucor miehei, Mucor pusillus, and Endothia parasitica. [, , , , ] These fungal-derived enzymes offer advantages like cost-effectiveness and suitability for vegetarian cheese production. [, , ]
Q8: What are the advantages of immobilizing Rennin for cheesemaking?
A: Immobilizing Rennin on various supports like sand offers several benefits, including reusability of the enzyme, potentially reducing production costs. [] Research has focused on optimizing the immobilization process to minimize enzyme leaching during cheese production. []
Q9: How does the specificity of Rennin from different sources compare?
A: Research comparing the specificity of calf Rennin to those derived from Mucor pusillus and Endothia parasitica reveals differences in the macropeptides released during casein hydrolysis. [] This suggests variations in their cleavage sites and potential impact on the final cheese characteristics. [, ]
Q10: Can Rennin be produced using recombinant DNA technology?
A: Yes, advancements in biotechnology have enabled the production of recombinant Rennin in various host organisms, including Escherichia coli, Saccharomyces cerevisiae, and Aspergillus species. [] This allows for a more sustainable and controlled production process, reducing the reliance on animal sources. []
Q11: What is the significance of the ratio of clotting activity (C) to proteolytic activity (P) in milk-clotting enzymes?
A: A high C/P ratio is desirable in cheesemaking as it ensures efficient milk clotting with minimal non-specific proteolysis, contributing to higher curd yields and desirable cheese characteristics. []
Q12: What are the future directions in Rennin research?
A: Ongoing research focuses on utilizing protein engineering techniques, like site-directed mutagenesis, to modify Rennin and enhance its properties. [] This includes improving its stability, altering its specificity, and potentially expanding its applications beyond cheesemaking. []
Disclaimer and Information on In-Vitro Research Products
Please be aware that all articles and product information presented on BenchChem are intended solely for informational purposes. The products available for purchase on BenchChem are specifically designed for in-vitro studies, which are conducted outside of living organisms. In-vitro studies, derived from the Latin term "in glass," involve experiments performed in controlled laboratory settings using cells or tissues. It is important to note that these products are not categorized as medicines or drugs, and they have not received approval from the FDA for the prevention, treatment, or cure of any medical condition, ailment, or disease. We must emphasize that any form of bodily introduction of these products into humans or animals is strictly prohibited by law. It is essential to adhere to these guidelines to ensure compliance with legal and ethical standards in research and experimentation.