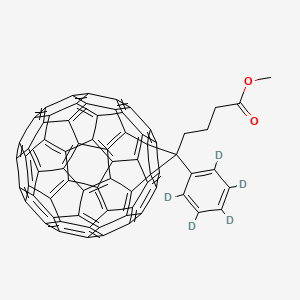
(6,6)-Pentadeuterophenyl C61 butyric AC&
Overview
Description
[6,6]-Pentadeuterophenyl C61 butyric acid methyl ester (PC60BM) is a deuterated fullerene that can be used as an acceptor molecule. It has a fullerene as the core and deuterium and benzyl as the attachment. The deuterium atoms facilitate an increase in the quantum efficiency of the electrochemical devices.
Mechanism of Action
Target of Action
The primary target of (6,6)-Pentadeuterophenyl C61 butyric acid methyl ester, also known as PCBM, is the electron transport layer in organic solar cells . It acts as an electron acceptor, facilitating the movement of electrons and enhancing the efficiency of these cells .
Mode of Action
PCBM interacts with its targets by accepting electrons from the photoactive layer in organic solar cells . This interaction results in the creation of an electric current, which is the primary function of solar cells .
Biochemical Pathways
The electron transport chain is a series of reactions that generate an electric current, and PCBM’s role as an electron acceptor is vital for this process .
Pharmacokinetics
It’s worth noting that pcbm has high electron mobility, which allows it to effectively accept and transport electrons .
Result of Action
The result of PCBM’s action is the generation of an electric current in organic solar cells . By accepting electrons from the photoactive layer, PCBM facilitates the movement of electrons, thereby generating an electric current .
Action Environment
The action of PCBM can be influenced by environmental factors. For instance, the formation of PCBM nanoscale aggregates using supercritical carbon dioxide (scCO2) annealing can improve the performance of bulk heterojunction solar cells .
Properties
InChI |
InChI=1S/C72H14O2/c1-74-11(73)8-5-9-70(10-6-3-2-4-7-10)71-66-58-50-40-30-22-14-12-13-16-20-18(14)26-34-28(20)38-32-24(16)25-17(13)21-19-15(12)23(22)31-37-27(19)35-29(21)39-33(25)43-42(32)52-46(38)56-48(34)54(44(50)36(26)30)62(66)64(56)68-60(52)61-53(43)47(39)57-49(35)55-45(37)51(41(31)40)59(58)67(71)63(55)65(57)69(61)72(68,70)71/h2-4,6-7H,5,8-9H2,1H3/i2D,3D,4D,6D,7D | |
---|---|---|
Details | Computed by InChI 1.0.6 (PubChem release 2021.05.07) | |
Source | PubChem | |
URL | https://pubchem.ncbi.nlm.nih.gov | |
Description | Data deposited in or computed by PubChem | |
InChI Key |
FIGVSQKKPIKBST-QRKCWBMQSA-N | |
Details | Computed by InChI 1.0.6 (PubChem release 2021.05.07) | |
Source | PubChem | |
URL | https://pubchem.ncbi.nlm.nih.gov | |
Description | Data deposited in or computed by PubChem | |
Canonical SMILES |
COC(=O)CCCC1(C23C14C5=C6C7=C8C9=C1C%10=C%11C%12=C%13C%14=C%10C%10=C1C1=C%15C%16=C%17C%18=C%19C%20=C%21C%22=C%23C%24=C%25C%26=C(C7=C9C%11=C%26C%12=C%24C%22=C%13C%20=C%14C%18=C%10%16)C7=C%25C9=C(C4=C76)C4=C2C(=C%17C3=C%15C5=C81)C%19=C%21C4=C%239)C1=CC=CC=C1 | |
Details | Computed by OEChem 2.3.0 (PubChem release 2021.05.07) | |
Source | PubChem | |
URL | https://pubchem.ncbi.nlm.nih.gov | |
Description | Data deposited in or computed by PubChem | |
Isomeric SMILES |
[2H]C1=C(C(=C(C(=C1[2H])[2H])C2(C34C25C6=C7C8=C9C1=C2C%10=C%11C%12=C%13C%14=C%10C%10=C2C2=C%15C%16=C%17C%18=C%19C%20=C%21C%22=C%23C%24=C%25C%26=C(C8=C1C%11=C%26C%12=C%24C%22=C%13C%20=C%14C%18=C%10%16)C1=C%25C8=C(C5=C17)C1=C3C(=C%17C4=C%15C6=C92)C%19=C%21C1=C%238)CCCC(=O)OC)[2H])[2H] | |
Details | Computed by OEChem 2.3.0 (PubChem release 2021.05.07) | |
Source | PubChem | |
URL | https://pubchem.ncbi.nlm.nih.gov | |
Description | Data deposited in or computed by PubChem | |
Molecular Formula |
C72H14O2 | |
Details | Computed by PubChem 2.1 (PubChem release 2021.05.07) | |
Source | PubChem | |
URL | https://pubchem.ncbi.nlm.nih.gov | |
Description | Data deposited in or computed by PubChem | |
Molecular Weight |
915.9 g/mol | |
Details | Computed by PubChem 2.1 (PubChem release 2021.05.07) | |
Source | PubChem | |
URL | https://pubchem.ncbi.nlm.nih.gov | |
Description | Data deposited in or computed by PubChem | |
CAS No. |
749898-80-4 | |
Record name | [6,6]-Pentadeuterophenyl C61 butyric acid methyl ester | |
Source | European Chemicals Agency (ECHA) | |
URL | https://echa.europa.eu/information-on-chemicals | |
Description | The European Chemicals Agency (ECHA) is an agency of the European Union which is the driving force among regulatory authorities in implementing the EU's groundbreaking chemicals legislation for the benefit of human health and the environment as well as for innovation and competitiveness. | |
Explanation | Use of the information, documents and data from the ECHA website is subject to the terms and conditions of this Legal Notice, and subject to other binding limitations provided for under applicable law, the information, documents and data made available on the ECHA website may be reproduced, distributed and/or used, totally or in part, for non-commercial purposes provided that ECHA is acknowledged as the source: "Source: European Chemicals Agency, http://echa.europa.eu/". Such acknowledgement must be included in each copy of the material. ECHA permits and encourages organisations and individuals to create links to the ECHA website under the following cumulative conditions: Links can only be made to webpages that provide a link to the Legal Notice page. | |
Retrosynthesis Analysis
AI-Powered Synthesis Planning: Our tool employs the Template_relevance Pistachio, Template_relevance Bkms_metabolic, Template_relevance Pistachio_ringbreaker, Template_relevance Reaxys, Template_relevance Reaxys_biocatalysis model, leveraging a vast database of chemical reactions to predict feasible synthetic routes.
One-Step Synthesis Focus: Specifically designed for one-step synthesis, it provides concise and direct routes for your target compounds, streamlining the synthesis process.
Accurate Predictions: Utilizing the extensive PISTACHIO, BKMS_METABOLIC, PISTACHIO_RINGBREAKER, REAXYS, REAXYS_BIOCATALYSIS database, our tool offers high-accuracy predictions, reflecting the latest in chemical research and data.
Strategy Settings
Precursor scoring | Relevance Heuristic |
---|---|
Min. plausibility | 0.01 |
Model | Template_relevance |
Template Set | Pistachio/Bkms_metabolic/Pistachio_ringbreaker/Reaxys/Reaxys_biocatalysis |
Top-N result to add to graph | 6 |
Feasible Synthetic Routes
Disclaimer and Information on In-Vitro Research Products
Please be aware that all articles and product information presented on BenchChem are intended solely for informational purposes. The products available for purchase on BenchChem are specifically designed for in-vitro studies, which are conducted outside of living organisms. In-vitro studies, derived from the Latin term "in glass," involve experiments performed in controlled laboratory settings using cells or tissues. It is important to note that these products are not categorized as medicines or drugs, and they have not received approval from the FDA for the prevention, treatment, or cure of any medical condition, ailment, or disease. We must emphasize that any form of bodily introduction of these products into humans or animals is strictly prohibited by law. It is essential to adhere to these guidelines to ensure compliance with legal and ethical standards in research and experimentation.