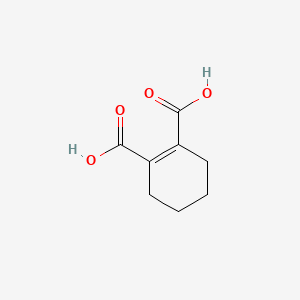
3,4,5,6-Tetrahydrophthalic acid
Overview
Description
3,4,5,6-Tetrahydrophthalic acid is an organic compound with the molecular formula C8H10O4. It is a derivative of phthalic acid and is characterized by the presence of a cyclohexene ring with two carboxylic acid groups. This compound is known for its applications in various chemical processes and industrial applications.
Preparation Methods
Synthetic Routes and Reaction Conditions: 3,4,5,6-Tetrahydrophthalic acid can be synthesized through the hydrogenation of phthalic anhydride. The process involves the use of a catalyst, typically palladium on carbon, under hydrogen gas at elevated pressures and temperatures. The reaction proceeds as follows: [ \text{C}_8\text{H}_4\text{O}_3 + 2\text{H}_2 \rightarrow \text{C}_8\text{H}_8\text{O}_3 ]
Industrial Production Methods: In industrial settings, this compound is often produced via the Diels-Alder reaction between maleic anhydride and butadiene, followed by hydrolysis of the resulting anhydride. This method is favored due to its efficiency and scalability.
Types of Reactions:
Oxidation: this compound can undergo oxidation reactions to form various oxidized derivatives.
Reduction: The compound can be reduced to form tetrahydrophthalic anhydride.
Substitution: It can participate in substitution reactions, particularly in the presence of nucleophiles.
Common Reagents and Conditions:
Oxidation: Common oxidizing agents include potassium permanganate and chromium trioxide.
Reduction: Hydrogen gas in the presence of a palladium catalyst is commonly used.
Substitution: Nucleophiles such as amines and alcohols can be used under mild conditions.
Major Products Formed:
Oxidation: Oxidized derivatives such as phthalic acid.
Reduction: Tetrahydrophthalic anhydride.
Substitution: Various substituted derivatives depending on the nucleophile used.
Scientific Research Applications
3,4,5,6-Tetrahydrophthalic acid has a wide range of applications in scientific research:
Chemistry: It is used as a building block for the synthesis of more complex organic molecules.
Biology: The compound is studied for its potential biological activities and interactions with biomolecules.
Medicine: Research is ongoing to explore its potential therapeutic applications.
Industry: It is used in the production of resins, coatings, and adhesives due to its chemical stability and reactivity.
Mechanism of Action
The mechanism of action of 3,4,5,6-Tetrahydrophthalic acid involves its interaction with various molecular targets. The compound can act as a ligand, binding to specific receptors or enzymes and modulating their activity. The pathways involved depend on the specific application and the biological system being studied.
Comparison with Similar Compounds
Phthalic acid: Similar in structure but lacks the cyclohexene ring.
Maleic acid: Contains a similar carboxylic acid functionality but differs in the overall structure.
Succinic acid: Another dicarboxylic acid with a simpler structure.
Uniqueness: 3,4,5,6-Tetrahydrophthalic acid is unique due to its cyclohexene ring structure, which imparts distinct chemical properties and reactivity compared to other dicarboxylic acids. This uniqueness makes it valuable in specific industrial and research applications.
Properties
IUPAC Name |
cyclohexene-1,2-dicarboxylic acid | |
---|---|---|
Details | Computed by Lexichem TK 2.7.0 (PubChem release 2021.05.07) | |
Source | PubChem | |
URL | https://pubchem.ncbi.nlm.nih.gov | |
Description | Data deposited in or computed by PubChem | |
InChI |
InChI=1S/C8H10O4/c9-7(10)5-3-1-2-4-6(5)8(11)12/h1-4H2,(H,9,10)(H,11,12) | |
Details | Computed by InChI 1.0.6 (PubChem release 2021.05.07) | |
Source | PubChem | |
URL | https://pubchem.ncbi.nlm.nih.gov | |
Description | Data deposited in or computed by PubChem | |
InChI Key |
UFDHBDMSHIXOKF-UHFFFAOYSA-N | |
Details | Computed by InChI 1.0.6 (PubChem release 2021.05.07) | |
Source | PubChem | |
URL | https://pubchem.ncbi.nlm.nih.gov | |
Description | Data deposited in or computed by PubChem | |
Canonical SMILES |
C1CCC(=C(C1)C(=O)O)C(=O)O | |
Details | Computed by OEChem 2.3.0 (PubChem release 2021.05.07) | |
Source | PubChem | |
URL | https://pubchem.ncbi.nlm.nih.gov | |
Description | Data deposited in or computed by PubChem | |
Molecular Formula |
C8H10O4 | |
Details | Computed by PubChem 2.1 (PubChem release 2021.05.07) | |
Source | PubChem | |
URL | https://pubchem.ncbi.nlm.nih.gov | |
Description | Data deposited in or computed by PubChem | |
DSSTOX Substance ID |
DTXSID10921878 | |
Record name | Cyclohex-1-ene-1,2-dicarboxylic acid | |
Source | EPA DSSTox | |
URL | https://comptox.epa.gov/dashboard/DTXSID10921878 | |
Description | DSSTox provides a high quality public chemistry resource for supporting improved predictive toxicology. | |
Molecular Weight |
170.16 g/mol | |
Details | Computed by PubChem 2.1 (PubChem release 2021.05.07) | |
Source | PubChem | |
URL | https://pubchem.ncbi.nlm.nih.gov | |
Description | Data deposited in or computed by PubChem | |
CAS No. |
635-08-5, 29965-78-4, 115959-69-8 | |
Record name | 1-Cyclohexene-1,2-dicarboxylic acid | |
Source | CAS Common Chemistry | |
URL | https://commonchemistry.cas.org/detail?cas_rn=635-08-5 | |
Description | CAS Common Chemistry is an open community resource for accessing chemical information. Nearly 500,000 chemical substances from CAS REGISTRY cover areas of community interest, including common and frequently regulated chemicals, and those relevant to high school and undergraduate chemistry classes. This chemical information, curated by our expert scientists, is provided in alignment with our mission as a division of the American Chemical Society. | |
Explanation | The data from CAS Common Chemistry is provided under a CC-BY-NC 4.0 license, unless otherwise stated. | |
Record name | 3,4,5,6-Tetrahydrophthalic acid | |
Source | ChemIDplus | |
URL | https://pubchem.ncbi.nlm.nih.gov/substance/?source=chemidplus&sourceid=0000635085 | |
Description | ChemIDplus is a free, web search system that provides access to the structure and nomenclature authority files used for the identification of chemical substances cited in National Library of Medicine (NLM) databases, including the TOXNET system. | |
Record name | Cyclohexene-1,2-dicarboxylic acid | |
Source | ChemIDplus | |
URL | https://pubchem.ncbi.nlm.nih.gov/substance/?source=chemidplus&sourceid=0029965784 | |
Description | ChemIDplus is a free, web search system that provides access to the structure and nomenclature authority files used for the identification of chemical substances cited in National Library of Medicine (NLM) databases, including the TOXNET system. | |
Record name | Cyclohex-1-ene-1,2-dicarboxylic acid | |
Source | EPA DSSTox | |
URL | https://comptox.epa.gov/dashboard/DTXSID10921878 | |
Description | DSSTox provides a high quality public chemistry resource for supporting improved predictive toxicology. | |
Record name | 3,4,5,6-tetrahydrophthalic acid | |
Source | European Chemicals Agency (ECHA) | |
URL | https://echa.europa.eu/substance-information/-/substanceinfo/100.010.205 | |
Description | The European Chemicals Agency (ECHA) is an agency of the European Union which is the driving force among regulatory authorities in implementing the EU's groundbreaking chemicals legislation for the benefit of human health and the environment as well as for innovation and competitiveness. | |
Explanation | Use of the information, documents and data from the ECHA website is subject to the terms and conditions of this Legal Notice, and subject to other binding limitations provided for under applicable law, the information, documents and data made available on the ECHA website may be reproduced, distributed and/or used, totally or in part, for non-commercial purposes provided that ECHA is acknowledged as the source: "Source: European Chemicals Agency, http://echa.europa.eu/". Such acknowledgement must be included in each copy of the material. ECHA permits and encourages organisations and individuals to create links to the ECHA website under the following cumulative conditions: Links can only be made to webpages that provide a link to the Legal Notice page. | |
Retrosynthesis Analysis
AI-Powered Synthesis Planning: Our tool employs the Template_relevance Pistachio, Template_relevance Bkms_metabolic, Template_relevance Pistachio_ringbreaker, Template_relevance Reaxys, Template_relevance Reaxys_biocatalysis model, leveraging a vast database of chemical reactions to predict feasible synthetic routes.
One-Step Synthesis Focus: Specifically designed for one-step synthesis, it provides concise and direct routes for your target compounds, streamlining the synthesis process.
Accurate Predictions: Utilizing the extensive PISTACHIO, BKMS_METABOLIC, PISTACHIO_RINGBREAKER, REAXYS, REAXYS_BIOCATALYSIS database, our tool offers high-accuracy predictions, reflecting the latest in chemical research and data.
Strategy Settings
Precursor scoring | Relevance Heuristic |
---|---|
Min. plausibility | 0.01 |
Model | Template_relevance |
Template Set | Pistachio/Bkms_metabolic/Pistachio_ringbreaker/Reaxys/Reaxys_biocatalysis |
Top-N result to add to graph | 6 |
Feasible Synthetic Routes
Disclaimer and Information on In-Vitro Research Products
Please be aware that all articles and product information presented on BenchChem are intended solely for informational purposes. The products available for purchase on BenchChem are specifically designed for in-vitro studies, which are conducted outside of living organisms. In-vitro studies, derived from the Latin term "in glass," involve experiments performed in controlled laboratory settings using cells or tissues. It is important to note that these products are not categorized as medicines or drugs, and they have not received approval from the FDA for the prevention, treatment, or cure of any medical condition, ailment, or disease. We must emphasize that any form of bodily introduction of these products into humans or animals is strictly prohibited by law. It is essential to adhere to these guidelines to ensure compliance with legal and ethical standards in research and experimentation.