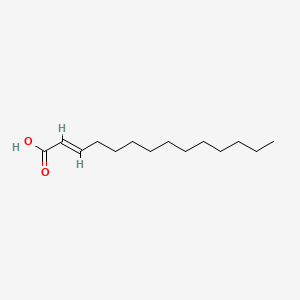
2-Tetradecenoic acid
Overview
Description
2-Tetradecenoic acid is a monounsaturated fatty acid with the molecular formula C14H26O2 It is characterized by a double bond located at the second carbon atom in the chain
Mechanism of Action
Biochemical Pathways
2-Tetradecenoic acid is likely involved in fatty acid biosynthesis . Fatty acids play crucial roles in numerous biochemical pathways, including energy production, inflammation, and cell signaling.
Action Environment
Environmental factors such as pH, temperature, and the presence of other molecules can influence the action, efficacy, and stability of this compound . For instance, its stability could be affected by exposure to heat or light, and its efficacy could be influenced by the presence of other fatty acids or metabolites.
Biochemical Analysis
Biochemical Properties
2-Tetradecenoic acid plays a crucial role in biochemical reactions, particularly in the biosynthesis of fatty acids. It is an intermediate in the fatty acid biosynthesis pathway, where it is converted from ®-3-Hydroxy-tetradecanoic acid via the action of enzymes such as fatty-acid synthase and 3-hydroxypalmitoyl . Additionally, this compound interacts with various proteins and enzymes, including dehydratase and thioesterase, which are involved in its biosynthesis and turnover .
Cellular Effects
This compound influences various cellular processes, including cell signaling pathways, gene expression, and cellular metabolism. In Gram-negative bacteria, it acts as a quorum sensing signal, regulating diverse biological functions . This compound can modulate virulence gene expression in response to environmental changes, highlighting its role in bacterial communication and pathogenicity . In mammalian cells, this compound is involved in lipid metabolism and can affect the expression of genes related to fatty acid biosynthesis .
Molecular Mechanism
At the molecular level, this compound exerts its effects through binding interactions with specific biomolecules. It can act as a ligand for certain receptors or enzymes, leading to the activation or inhibition of these targets. For example, in bacterial quorum sensing systems, this compound binds to receptor proteins, triggering a cascade of signaling events that regulate gene expression . Additionally, it may influence enzyme activity by acting as a substrate or inhibitor, thereby modulating metabolic pathways .
Temporal Effects in Laboratory Settings
In laboratory settings, the effects of this compound can change over time due to its stability and degradation. Studies have shown that this compound can be stable under certain conditions, but it may degrade over time, leading to changes in its biological activity . Long-term exposure to this compound in in vitro or in vivo studies has revealed its potential impact on cellular function, including alterations in gene expression and metabolic processes .
Dosage Effects in Animal Models
The effects of this compound vary with different dosages in animal models. At low doses, it may exhibit beneficial effects, such as modulation of lipid metabolism and anti-inflammatory properties . At high doses, this compound can have toxic or adverse effects, including hepatotoxicity and disruption of normal cellular functions . Threshold effects have been observed, indicating that the compound’s impact is dose-dependent.
Metabolic Pathways
This compound is involved in several metabolic pathways, including fatty acid biosynthesis and degradation. It interacts with enzymes such as fatty-acid synthase and 3-hydroxypalmitoyl, which facilitate its conversion and utilization in metabolic processes . This compound can also affect metabolic flux and metabolite levels, influencing overall cellular metabolism .
Transport and Distribution
Within cells and tissues, this compound is transported and distributed through interactions with specific transporters and binding proteins. These interactions facilitate its localization and accumulation in various cellular compartments . The compound’s distribution can impact its biological activity and effectiveness in different tissues .
Subcellular Localization
This compound’s subcellular localization is influenced by targeting signals and post-translational modifications. It can be directed to specific compartments or organelles, where it exerts its effects on cellular functions . Understanding the subcellular localization of this compound is crucial for elucidating its role in cellular processes and its potential therapeutic applications .
Preparation Methods
Synthetic Routes and Reaction Conditions: 2-Tetradecenoic acid can be synthesized through several methods. One common approach involves the catalytic hydrogenation of tetradecynoic acid, which introduces a double bond at the desired position. Another method includes the use of Grignard reagents, where a halogenated tetradecane reacts with magnesium to form a Grignard reagent, which is then treated with carbon dioxide to yield the acid.
Industrial Production Methods: In industrial settings, this compound is often produced through the hydrolysis of triglycerides found in natural oils. This process involves the saponification of fats and oils, followed by the selective hydrogenation of the resulting fatty acids to introduce the double bond at the second carbon position.
Chemical Reactions Analysis
Types of Reactions: 2-Tetradecenoic acid undergoes various chemical reactions, including:
Oxidation: This reaction typically involves the use of oxidizing agents like potassium permanganate or ozone, leading to the formation of shorter-chain carboxylic acids.
Reduction: Catalytic hydrogenation can reduce the double bond, converting this compound into tetradecanoic acid.
Substitution: Halogenation reactions can introduce halogen atoms at specific positions along the carbon chain.
Common Reagents and Conditions:
Oxidation: Potassium permanganate in an alkaline medium.
Reduction: Hydrogen gas in the presence of a palladium or platinum catalyst.
Substitution: Halogenation using bromine or chlorine in the presence of a catalyst like iron.
Major Products Formed:
Oxidation: Shorter-chain carboxylic acids.
Reduction: Tetradecanoic acid.
Substitution: Halogenated tetradecenoic acids.
Scientific Research Applications
2-Tetradecenoic acid has a wide range of applications in scientific research:
Chemistry: It is used as a precursor in the synthesis of various organic compounds and as a standard in chromatographic analyses.
Biology: This compound is studied for its role in cellular metabolism and as a signaling molecule in various biological pathways.
Medicine: Research has explored its potential as an anti-inflammatory agent and its effects on lipid metabolism.
Industry: It is utilized in the production of surfactants, lubricants, and as an intermediate in the synthesis of other chemicals.
Comparison with Similar Compounds
Tetradecanoic acid (Myristic acid): A saturated fatty acid with no double bonds.
Oleic acid: A monounsaturated fatty acid with a double bond at the ninth carbon position.
Palmitoleic acid: A monounsaturated fatty acid with a double bond at the seventh carbon position.
Uniqueness: 2-Tetradecenoic acid is unique due to its specific double bond position at the second carbon, which imparts distinct chemical and biological properties. This structural feature differentiates it from other monounsaturated fatty acids and influences its reactivity and function in various applications.
Properties
CAS No. |
39525-69-4 |
---|---|
Molecular Formula |
C14H26O2 |
Molecular Weight |
226.35 g/mol |
IUPAC Name |
tetradec-2-enoic acid |
InChI |
InChI=1S/C14H26O2/c1-2-3-4-5-6-7-8-9-10-11-12-13-14(15)16/h12-13H,2-11H2,1H3,(H,15,16) |
InChI Key |
IBYFOBGPNPINBU-UHFFFAOYSA-N |
Isomeric SMILES |
CCCCCCCCCCC/C=C/C(=O)O |
SMILES |
CCCCCCCCCCCC=CC(=O)O |
Canonical SMILES |
CCCCCCCCCCCC=CC(=O)O |
physical_description |
Solid |
Origin of Product |
United States |
Retrosynthesis Analysis
AI-Powered Synthesis Planning: Our tool employs the Template_relevance Pistachio, Template_relevance Bkms_metabolic, Template_relevance Pistachio_ringbreaker, Template_relevance Reaxys, Template_relevance Reaxys_biocatalysis model, leveraging a vast database of chemical reactions to predict feasible synthetic routes.
One-Step Synthesis Focus: Specifically designed for one-step synthesis, it provides concise and direct routes for your target compounds, streamlining the synthesis process.
Accurate Predictions: Utilizing the extensive PISTACHIO, BKMS_METABOLIC, PISTACHIO_RINGBREAKER, REAXYS, REAXYS_BIOCATALYSIS database, our tool offers high-accuracy predictions, reflecting the latest in chemical research and data.
Strategy Settings
Precursor scoring | Relevance Heuristic |
---|---|
Min. plausibility | 0.01 |
Model | Template_relevance |
Template Set | Pistachio/Bkms_metabolic/Pistachio_ringbreaker/Reaxys/Reaxys_biocatalysis |
Top-N result to add to graph | 6 |
Feasible Synthetic Routes
Q1: How does tetradecenoic acid function as a signaling molecule in bacteria?
A1: Tetradecenoic acid acts as a diffusible signal factor (DSF) in certain bacteria like Xylella fastidiosa. [, ] It is produced by the enzyme RpfF and accumulates as cell density increases. [] This accumulation triggers changes in gene expression, impacting bacterial behaviors such as biofilm formation, cell adhesion, and virulence. [, ]
Q2: How does tetradecenoic acid impact the virulence of Xylella fastidiosa?
A3: Studies have shown that mutants of X. fastidiosa unable to produce tetradecenoic acid exhibit increased virulence in grapes. [] This suggests that the accumulation of tetradecenoic acid typically suppresses virulence. Artificially increasing DSF levels in plants could therefore be a potential strategy for disease control, a concept called "pathogen confusion." []
Q3: What is the molecular formula and weight of tetradecenoic acid?
A3: The molecular formula of tetradecenoic acid is C14H26O2, and its molecular weight is 226.36 g/mol.
Q4: How are different isomers of tetradecenoic acid distinguished?
A5: Isomers of tetradecenoic acid differ in the position and geometry of the double bond. Techniques like gas chromatography-mass spectrometry (GC-MS) are crucial for identifying and separating these isomers based on their unique retention times and fragmentation patterns. [, ]
Q5: Is tetradecenoic acid metabolized in mammals?
A7: Research on the closely related compound, nitro-9-cis-octadecenoic acid (18:1-NO2), suggests that unsaturated fatty acids like tetradecenoic acid can undergo β-oxidation in the liver. [] This process results in shorter-chain nitro-fatty acids as metabolites. [] Further research is needed to confirm if tetradecenoic acid undergoes a similar metabolic pathway.
Q6: How is tetradecenoic acid incorporated into biological systems?
A8: In certain organisms, tetradecenoic acid is incorporated into complex lipids like phospholipids. [] For instance, the unusual 7-methyl-6-octadecenoic acid, structurally similar to tetradecenoic acid, was discovered in the phospholipids of the sea cucumber Holothuria mexicana. []
Q7: Can tetradecenoic acid interact with proteins?
A9: While direct interaction studies with tetradecenoic acid are limited, research on nitro-fatty acids shows that they readily form adducts with thiol-containing proteins and glutathione in vivo. [] This suggests that tetradecenoic acid, with its unsaturated structure, could potentially interact with proteins through similar mechanisms.
Q8: How is tetradecenoic acid typically analyzed in biological samples?
A10: GC-MS is a widely used technique for analyzing tetradecenoic acid in biological samples. [, , ] This method allows for the separation and identification of different isomers based on their unique retention times and mass spectra.
Q9: Are there challenges in accurately quantifying tetradecenoic acid?
A11: Quantifying tetradecenoic acid, especially in low concentrations, can be challenging due to its potential to co-elute with other fatty acids during GC analysis. [] For accurate quantification, meticulous optimization of GC conditions is essential to ensure clear separation from other components. []
Disclaimer and Information on In-Vitro Research Products
Please be aware that all articles and product information presented on BenchChem are intended solely for informational purposes. The products available for purchase on BenchChem are specifically designed for in-vitro studies, which are conducted outside of living organisms. In-vitro studies, derived from the Latin term "in glass," involve experiments performed in controlled laboratory settings using cells or tissues. It is important to note that these products are not categorized as medicines or drugs, and they have not received approval from the FDA for the prevention, treatment, or cure of any medical condition, ailment, or disease. We must emphasize that any form of bodily introduction of these products into humans or animals is strictly prohibited by law. It is essential to adhere to these guidelines to ensure compliance with legal and ethical standards in research and experimentation.