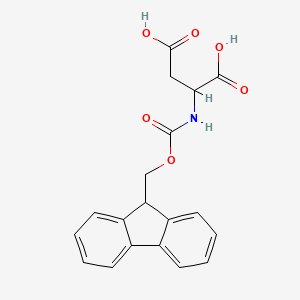
Fmoc-DL-aspartic acid
Overview
Description
Fmoc-DL-aspartic acid is a useful research compound. Its molecular formula is C19H17NO6 and its molecular weight is 355.3 g/mol. The purity is usually 95%.
The exact mass of the compound this compound is 355.10558726 g/mol and the complexity rating of the compound is 528. The storage condition is unknown. Please store according to label instructions upon receipt of goods.
BenchChem offers high-quality this compound suitable for many research applications. Different packaging options are available to accommodate customers' requirements. Please inquire for more information about this compound including the price, delivery time, and more detailed information at info@benchchem.com.
Mechanism of Action
Target of Action
Fmoc-DL-aspartic acid, also known as 2-{[(9H-fluoren-9-ylmethoxy)carbonyl]amino}butanedioic acid, is primarily used as a building block in the synthesis of peptides . The primary targets of this compound are the amino acids that make up peptide chains .
Mode of Action
The Fmoc group acts as a protective group for amines during peptide synthesis . It can be introduced by reacting the amine with fluorenylmethyloxycarbonyl chloride (Fmoc-Cl) . The Fmoc group is base-labile, meaning it can be removed under basic conditions, allowing the exposed amine to react with the next amino acid in the peptide sequence .
Biochemical Pathways
This compound plays a crucial role in the solid-phase peptide synthesis (SPPS) pathway . In this process, the Fmoc group protects the amine group of the amino acid during the elongation of the peptide chain . Once the peptide bond is formed, the Fmoc group is removed, allowing the next amino acid to be added to the growing peptide chain .
Result of Action
The primary result of the action of this compound is the successful synthesis of peptides . By protecting the amine group during synthesis, it allows for the controlled formation of peptide bonds, leading to the creation of specific peptide sequences .
Action Environment
The action of this compound is highly dependent on the environment in which the peptide synthesis takes place. Factors such as temperature, pH, and the presence of other reactants can all influence the efficacy and stability of the compound . For example, the removal of the Fmoc group requires basic conditions . Therefore, the pH of the environment is a critical factor in the compound’s action.
Biochemical Analysis
Biochemical Properties
Fmoc-DL-aspartic acid is known for its self-assembly features, which are due to the inherent hydrophobicity and aromaticity of the Fmoc moiety . This property allows it to interact with various enzymes, proteins, and other biomolecules, promoting the association of building blocks in biochemical reactions .
Cellular Effects
This compound has been shown to have effects on various types of cells and cellular processes. For instance, it has been used in the synthesis of aggregation-prone protein domains, indicating its potential role in influencing cell function .
Molecular Mechanism
The molecular mechanism of this compound involves its role as a protecting group in organic synthesis . It is introduced by reacting the amine with fluorenylmethyloxycarbonyl chloride (Fmoc-Cl), and is rapidly removed by base . This process allows for the controlled synthesis of complex molecules, including peptides .
Temporal Effects in Laboratory Settings
In laboratory settings, this compound has been used in solid-phase peptide synthesis (SPPS), a method for producing peptides . Over time, the compound’s stability and degradation can influence the efficiency of the synthesis process .
Dosage Effects in Animal Models
While specific studies on the dosage effects of this compound in animal models are limited, it is known that D-aspartic acid, the precursor of this compound, plays a significant role in the reproduction of vertebrates
Metabolic Pathways
Aspartate, the core component of this compound, is involved in several metabolic pathways. It is the common precursor of the essential amino acids lysine, threonine, methionine, and isoleucine in higher plants . Additionally, aspartate can be converted to asparagine .
Subcellular Localization
Given its role in peptide synthesis, it is likely to be found in areas of the cell where protein synthesis occurs, such as the cytoplasm and the endoplasmic reticulum .
Properties
IUPAC Name |
2-(9H-fluoren-9-ylmethoxycarbonylamino)butanedioic acid | |
---|---|---|
Details | Computed by Lexichem TK 2.7.0 (PubChem release 2021.05.07) | |
Source | PubChem | |
URL | https://pubchem.ncbi.nlm.nih.gov | |
Description | Data deposited in or computed by PubChem | |
InChI |
InChI=1S/C19H17NO6/c21-17(22)9-16(18(23)24)20-19(25)26-10-15-13-7-3-1-5-11(13)12-6-2-4-8-14(12)15/h1-8,15-16H,9-10H2,(H,20,25)(H,21,22)(H,23,24) | |
Details | Computed by InChI 1.0.6 (PubChem release 2021.05.07) | |
Source | PubChem | |
URL | https://pubchem.ncbi.nlm.nih.gov | |
Description | Data deposited in or computed by PubChem | |
InChI Key |
KSDTXRUIZMTBNV-UHFFFAOYSA-N | |
Details | Computed by InChI 1.0.6 (PubChem release 2021.05.07) | |
Source | PubChem | |
URL | https://pubchem.ncbi.nlm.nih.gov | |
Description | Data deposited in or computed by PubChem | |
Canonical SMILES |
C1=CC=C2C(=C1)C(C3=CC=CC=C32)COC(=O)NC(CC(=O)O)C(=O)O | |
Details | Computed by OEChem 2.3.0 (PubChem release 2021.05.07) | |
Source | PubChem | |
URL | https://pubchem.ncbi.nlm.nih.gov | |
Description | Data deposited in or computed by PubChem | |
Molecular Formula |
C19H17NO6 | |
Details | Computed by PubChem 2.1 (PubChem release 2021.05.07) | |
Source | PubChem | |
URL | https://pubchem.ncbi.nlm.nih.gov | |
Description | Data deposited in or computed by PubChem | |
Molecular Weight |
355.3 g/mol | |
Details | Computed by PubChem 2.1 (PubChem release 2021.05.07) | |
Source | PubChem | |
URL | https://pubchem.ncbi.nlm.nih.gov | |
Description | Data deposited in or computed by PubChem | |
CAS No. |
136083-73-3, 286460-78-4 | |
Record name | N-[(9H-Fluoren-9-ylmethoxy)carbonyl]aspartic acid | |
Source | CAS Common Chemistry | |
URL | https://commonchemistry.cas.org/detail?cas_rn=136083-73-3 | |
Description | CAS Common Chemistry is an open community resource for accessing chemical information. Nearly 500,000 chemical substances from CAS REGISTRY cover areas of community interest, including common and frequently regulated chemicals, and those relevant to high school and undergraduate chemistry classes. This chemical information, curated by our expert scientists, is provided in alignment with our mission as a division of the American Chemical Society. | |
Explanation | The data from CAS Common Chemistry is provided under a CC-BY-NC 4.0 license, unless otherwise stated. | |
Record name | N-(9-Fluorenylmethoxycarbonyl)-L-aspartic-2-13C acid | |
Source | European Chemicals Agency (ECHA) | |
URL | https://echa.europa.eu/information-on-chemicals | |
Description | The European Chemicals Agency (ECHA) is an agency of the European Union which is the driving force among regulatory authorities in implementing the EU's groundbreaking chemicals legislation for the benefit of human health and the environment as well as for innovation and competitiveness. | |
Explanation | Use of the information, documents and data from the ECHA website is subject to the terms and conditions of this Legal Notice, and subject to other binding limitations provided for under applicable law, the information, documents and data made available on the ECHA website may be reproduced, distributed and/or used, totally or in part, for non-commercial purposes provided that ECHA is acknowledged as the source: "Source: European Chemicals Agency, http://echa.europa.eu/". Such acknowledgement must be included in each copy of the material. ECHA permits and encourages organisations and individuals to create links to the ECHA website under the following cumulative conditions: Links can only be made to webpages that provide a link to the Legal Notice page. | |
Retrosynthesis Analysis
AI-Powered Synthesis Planning: Our tool employs the Template_relevance Pistachio, Template_relevance Bkms_metabolic, Template_relevance Pistachio_ringbreaker, Template_relevance Reaxys, Template_relevance Reaxys_biocatalysis model, leveraging a vast database of chemical reactions to predict feasible synthetic routes.
One-Step Synthesis Focus: Specifically designed for one-step synthesis, it provides concise and direct routes for your target compounds, streamlining the synthesis process.
Accurate Predictions: Utilizing the extensive PISTACHIO, BKMS_METABOLIC, PISTACHIO_RINGBREAKER, REAXYS, REAXYS_BIOCATALYSIS database, our tool offers high-accuracy predictions, reflecting the latest in chemical research and data.
Strategy Settings
Precursor scoring | Relevance Heuristic |
---|---|
Min. plausibility | 0.01 |
Model | Template_relevance |
Template Set | Pistachio/Bkms_metabolic/Pistachio_ringbreaker/Reaxys/Reaxys_biocatalysis |
Top-N result to add to graph | 6 |
Feasible Synthetic Routes
Disclaimer and Information on In-Vitro Research Products
Please be aware that all articles and product information presented on BenchChem are intended solely for informational purposes. The products available for purchase on BenchChem are specifically designed for in-vitro studies, which are conducted outside of living organisms. In-vitro studies, derived from the Latin term "in glass," involve experiments performed in controlled laboratory settings using cells or tissues. It is important to note that these products are not categorized as medicines or drugs, and they have not received approval from the FDA for the prevention, treatment, or cure of any medical condition, ailment, or disease. We must emphasize that any form of bodily introduction of these products into humans or animals is strictly prohibited by law. It is essential to adhere to these guidelines to ensure compliance with legal and ethical standards in research and experimentation.