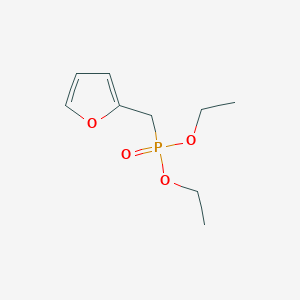
2-(diethoxyphosphorylmethyl)furan
Overview
Description
2-(Diethoxyphosphorylmethyl)furan is an organic compound that belongs to the class of furan derivatives. Furans are heterocyclic aromatic compounds characterized by a five-membered ring containing one oxygen atom. The presence of the diethoxyphosphorylmethyl group in this compound introduces unique chemical properties, making it a subject of interest in various fields of scientific research.
Preparation Methods
Synthetic Routes and Reaction Conditions
The synthesis of 2-(diethoxyphosphorylmethyl)furan typically involves the bromination of the corresponding (diethoxyphosphorylmethyl)furans or a multi-step synthesis starting from isomeric methyl-furancarboxylates. The process includes bromination, reduction to the corresponding alcohols, substitution of the hydroxy group with halogen, and phosphorylation by the Michaelis-Becker reaction .
Industrial Production Methods
Industrial production methods for this compound are not extensively documented. the general approach involves large-scale synthesis using the aforementioned synthetic routes, with optimization for yield and purity through controlled reaction conditions and purification techniques.
Chemical Reactions Analysis
Types of Reactions
2-(Diethoxyphosphorylmethyl)furan undergoes various chemical reactions, including:
Oxidation: The compound can be oxidized under specific conditions to form corresponding oxidized derivatives.
Reduction: Reduction reactions can convert the compound into different reduced forms.
Substitution: The compound can undergo substitution reactions, where functional groups are replaced by other groups.
Common Reagents and Conditions
Common reagents used in these reactions include bromine, dioxane dibromide, chloroform, acetic acid, and hydrogen bromide . Reaction conditions vary depending on the desired product, with temperature, solvent, and catalyst playing crucial roles.
Major Products Formed
Major products formed from these reactions include bromoacetyl derivatives, furylpyrazines, and phosphorylated furyl carbonates .
Scientific Research Applications
2-(Diethoxyphosphorylmethyl)furan has several scientific research applications:
Mechanism of Action
The mechanism of action of 2-(diethoxyphosphorylmethyl)furan involves its interaction with specific molecular targets and pathways. For instance, in the Curtius reaction, the compound undergoes hydrolysis, rearrangement, and nucleophilic substitution to form various products . The detailed molecular pathways and targets depend on the specific application and reaction conditions.
Comparison with Similar Compounds
Similar Compounds
Similar compounds to 2-(diethoxyphosphorylmethyl)furan include:
- 3-(Diethoxyphosphorylmethyl)-2-furoyl azide
- 5-(Diethoxyphosphorylmethyl)-2-furoyl azide
- 3-Methoxymethyl-2-furoates
- 3-Chloromethyl-2-furoates
Uniqueness
This compound is unique due to its specific diethoxyphosphorylmethyl group, which imparts distinct chemical properties and reactivity. This uniqueness makes it valuable in specialized synthetic applications and research studies.
Properties
IUPAC Name |
2-(diethoxyphosphorylmethyl)furan | |
---|---|---|
Details | Computed by LexiChem 2.6.6 (PubChem release 2019.06.18) | |
Source | PubChem | |
URL | https://pubchem.ncbi.nlm.nih.gov | |
Description | Data deposited in or computed by PubChem | |
InChI |
InChI=1S/C9H15O4P/c1-3-12-14(10,13-4-2)8-9-6-5-7-11-9/h5-7H,3-4,8H2,1-2H3 | |
Details | Computed by InChI 1.0.5 (PubChem release 2019.06.18) | |
Source | PubChem | |
URL | https://pubchem.ncbi.nlm.nih.gov | |
Description | Data deposited in or computed by PubChem | |
InChI Key |
PSJWVTYRXDJTFC-UHFFFAOYSA-N | |
Details | Computed by InChI 1.0.5 (PubChem release 2019.06.18) | |
Source | PubChem | |
URL | https://pubchem.ncbi.nlm.nih.gov | |
Description | Data deposited in or computed by PubChem | |
Canonical SMILES |
CCOP(=O)(CC1=CC=CO1)OCC | |
Details | Computed by OEChem 2.1.5 (PubChem release 2019.06.18) | |
Source | PubChem | |
URL | https://pubchem.ncbi.nlm.nih.gov | |
Description | Data deposited in or computed by PubChem | |
Molecular Formula |
C9H15O4P | |
Details | Computed by PubChem 2.1 (PubChem release 2019.06.18) | |
Source | PubChem | |
URL | https://pubchem.ncbi.nlm.nih.gov | |
Description | Data deposited in or computed by PubChem | |
DSSTOX Substance ID |
DTXSID90364643 | |
Record name | Phosphonic acid, (2-furanylmethyl)-, diethyl ester | |
Source | EPA DSSTox | |
URL | https://comptox.epa.gov/dashboard/DTXSID90364643 | |
Description | DSSTox provides a high quality public chemistry resource for supporting improved predictive toxicology. | |
Molecular Weight |
218.19 g/mol | |
Details | Computed by PubChem 2.1 (PubChem release 2021.05.07) | |
Source | PubChem | |
URL | https://pubchem.ncbi.nlm.nih.gov | |
Description | Data deposited in or computed by PubChem | |
CAS No. |
39996-86-6 | |
Record name | Phosphonic acid, (2-furanylmethyl)-, diethyl ester | |
Source | EPA DSSTox | |
URL | https://comptox.epa.gov/dashboard/DTXSID90364643 | |
Description | DSSTox provides a high quality public chemistry resource for supporting improved predictive toxicology. | |
Retrosynthesis Analysis
AI-Powered Synthesis Planning: Our tool employs the Template_relevance Pistachio, Template_relevance Bkms_metabolic, Template_relevance Pistachio_ringbreaker, Template_relevance Reaxys, Template_relevance Reaxys_biocatalysis model, leveraging a vast database of chemical reactions to predict feasible synthetic routes.
One-Step Synthesis Focus: Specifically designed for one-step synthesis, it provides concise and direct routes for your target compounds, streamlining the synthesis process.
Accurate Predictions: Utilizing the extensive PISTACHIO, BKMS_METABOLIC, PISTACHIO_RINGBREAKER, REAXYS, REAXYS_BIOCATALYSIS database, our tool offers high-accuracy predictions, reflecting the latest in chemical research and data.
Strategy Settings
Precursor scoring | Relevance Heuristic |
---|---|
Min. plausibility | 0.01 |
Model | Template_relevance |
Template Set | Pistachio/Bkms_metabolic/Pistachio_ringbreaker/Reaxys/Reaxys_biocatalysis |
Top-N result to add to graph | 6 |
Feasible Synthetic Routes
Disclaimer and Information on In-Vitro Research Products
Please be aware that all articles and product information presented on BenchChem are intended solely for informational purposes. The products available for purchase on BenchChem are specifically designed for in-vitro studies, which are conducted outside of living organisms. In-vitro studies, derived from the Latin term "in glass," involve experiments performed in controlled laboratory settings using cells or tissues. It is important to note that these products are not categorized as medicines or drugs, and they have not received approval from the FDA for the prevention, treatment, or cure of any medical condition, ailment, or disease. We must emphasize that any form of bodily introduction of these products into humans or animals is strictly prohibited by law. It is essential to adhere to these guidelines to ensure compliance with legal and ethical standards in research and experimentation.