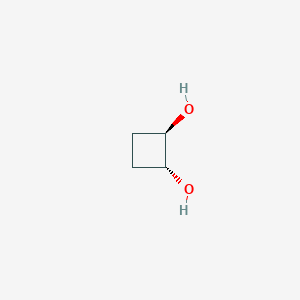
trans-Cyclobutane-1,2-diol
Overview
Description
trans-Cyclobutane-1,2-diol: is an organic compound with the molecular formula C4H8O2. It is a diol, meaning it contains two hydroxyl groups (-OH) attached to a cyclobutane ring. The trans configuration indicates that the hydroxyl groups are on opposite sides of the cyclobutane ring, giving the molecule a unique three-dimensional structure. This compound is of interest in various fields of chemistry due to its strained ring system and potential for diverse chemical reactions.
Preparation Methods
Synthetic Routes and Reaction Conditions: trans-Cyclobutane-1,2-diol can be synthesized through several methods. One common approach involves the nucleophilic addition of organolithium or Grignard reagents to 2-hydroxycyclobutanone, followed by reduction . Another method includes the photodimerization of trans-cinnamic acid, which forms a cyclobutane ring .
Industrial Production Methods: While specific industrial production methods for this compound are not well-documented, the synthesis typically involves scalable reactions such as the use of organolithium reagents and photodimerization techniques. These methods can be adapted for larger-scale production with appropriate optimization of reaction conditions.
Chemical Reactions Analysis
Types of Reactions: trans-Cyclobutane-1,2-diol undergoes various chemical reactions, including:
Reduction: Reduction of this compound can yield cyclobutane derivatives with different functional groups.
Substitution: The hydroxyl groups can be substituted with other functional groups through reactions with appropriate reagents.
Common Reagents and Conditions:
Oxidation: DMSO, dioxomolybdenum(VI) catalysts.
Reduction: Metal hydrides or catalytic hydrogenation.
Substitution: Halogenating agents, sulfonyl chlorides.
Major Products:
Oxidation: 1,4-dicarbonyl compounds.
Reduction: Cyclobutane derivatives with modified functional groups.
Substitution: Cyclobutane derivatives with substituted functional groups.
Scientific Research Applications
Chemistry: trans-Cyclobutane-1,2-diol is used as a building block in organic synthesis. Its strained ring system makes it a valuable intermediate for the synthesis of complex molecules, including natural products and pharmaceuticals .
Biology and Medicine: The compound’s unique structure allows it to interact with biological molecules in specific ways, making it a potential candidate for drug development and biochemical studies .
Industry: In the industrial sector, this compound can be used in the synthesis of polymers and other materials that require rigid, strained ring systems for enhanced properties .
Mechanism of Action
The mechanism by which trans-cyclobutane-1,2-diol exerts its effects depends on the specific reaction or application. In oxidation reactions, the hydroxyl groups are typically targeted by oxidizing agents, leading to the formation of carbonyl compounds . The strained ring system of cyclobutane allows for unique reactivity patterns, facilitating various chemical transformations.
Comparison with Similar Compounds
cis-Cyclobutane-1,2-diol: The cis isomer has hydroxyl groups on the same side of the cyclobutane ring, leading to different chemical and physical properties.
Cyclobutane-1,2-dione: This compound has two carbonyl groups instead of hydroxyl groups, resulting in distinct reactivity.
Cyclobutane-1,2-dicarboxylic acid: Contains carboxyl groups, making it more acidic and reactive in different chemical contexts.
Uniqueness: trans-Cyclobutane-1,2-diol’s trans configuration and diol functionality make it unique among cyclobutane derivatives.
Properties
IUPAC Name |
(1R,2R)-cyclobutane-1,2-diol | |
---|---|---|
Details | Computed by LexiChem 2.6.6 (PubChem release 2019.06.18) | |
Source | PubChem | |
URL | https://pubchem.ncbi.nlm.nih.gov | |
Description | Data deposited in or computed by PubChem | |
InChI |
InChI=1S/C4H8O2/c5-3-1-2-4(3)6/h3-6H,1-2H2/t3-,4-/m1/s1 | |
Details | Computed by InChI 1.0.5 (PubChem release 2019.06.18) | |
Source | PubChem | |
URL | https://pubchem.ncbi.nlm.nih.gov | |
Description | Data deposited in or computed by PubChem | |
InChI Key |
MHPMXFUDCYMCOE-QWWZWVQMSA-N | |
Details | Computed by InChI 1.0.5 (PubChem release 2019.06.18) | |
Source | PubChem | |
URL | https://pubchem.ncbi.nlm.nih.gov | |
Description | Data deposited in or computed by PubChem | |
Canonical SMILES |
C1CC(C1O)O | |
Details | Computed by OEChem 2.1.5 (PubChem release 2019.06.18) | |
Source | PubChem | |
URL | https://pubchem.ncbi.nlm.nih.gov | |
Description | Data deposited in or computed by PubChem | |
Isomeric SMILES |
C1C[C@H]([C@@H]1O)O | |
Details | Computed by OEChem 2.1.5 (PubChem release 2019.06.18) | |
Source | PubChem | |
URL | https://pubchem.ncbi.nlm.nih.gov | |
Description | Data deposited in or computed by PubChem | |
Molecular Formula |
C4H8O2 | |
Details | Computed by PubChem 2.1 (PubChem release 2019.06.18) | |
Source | PubChem | |
URL | https://pubchem.ncbi.nlm.nih.gov | |
Description | Data deposited in or computed by PubChem | |
Molecular Weight |
88.11 g/mol | |
Details | Computed by PubChem 2.1 (PubChem release 2021.05.07) | |
Source | PubChem | |
URL | https://pubchem.ncbi.nlm.nih.gov | |
Description | Data deposited in or computed by PubChem | |
Retrosynthesis Analysis
AI-Powered Synthesis Planning: Our tool employs the Template_relevance Pistachio, Template_relevance Bkms_metabolic, Template_relevance Pistachio_ringbreaker, Template_relevance Reaxys, Template_relevance Reaxys_biocatalysis model, leveraging a vast database of chemical reactions to predict feasible synthetic routes.
One-Step Synthesis Focus: Specifically designed for one-step synthesis, it provides concise and direct routes for your target compounds, streamlining the synthesis process.
Accurate Predictions: Utilizing the extensive PISTACHIO, BKMS_METABOLIC, PISTACHIO_RINGBREAKER, REAXYS, REAXYS_BIOCATALYSIS database, our tool offers high-accuracy predictions, reflecting the latest in chemical research and data.
Strategy Settings
Precursor scoring | Relevance Heuristic |
---|---|
Min. plausibility | 0.01 |
Model | Template_relevance |
Template Set | Pistachio/Bkms_metabolic/Pistachio_ringbreaker/Reaxys/Reaxys_biocatalysis |
Top-N result to add to graph | 6 |
Feasible Synthetic Routes
Disclaimer and Information on In-Vitro Research Products
Please be aware that all articles and product information presented on BenchChem are intended solely for informational purposes. The products available for purchase on BenchChem are specifically designed for in-vitro studies, which are conducted outside of living organisms. In-vitro studies, derived from the Latin term "in glass," involve experiments performed in controlled laboratory settings using cells or tissues. It is important to note that these products are not categorized as medicines or drugs, and they have not received approval from the FDA for the prevention, treatment, or cure of any medical condition, ailment, or disease. We must emphasize that any form of bodily introduction of these products into humans or animals is strictly prohibited by law. It is essential to adhere to these guidelines to ensure compliance with legal and ethical standards in research and experimentation.