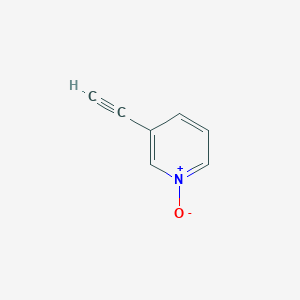
3-Ethynyl-pyridine 1-oxide
Overview
Description
3-Ethynyl-pyridine 1-oxide is an organic compound with the molecular formula C7H5NO It is a derivative of pyridine, where the hydrogen atom at the third position is replaced by an ethynyl group, and the nitrogen atom is oxidized to form an N-oxide
Preparation Methods
Synthetic Routes and Reaction Conditions
The synthesis of 3-Ethynyl-pyridine 1-oxide typically involves the oxidation of 3-Ethynyl-pyridine. One common method is the use of hydrogen peroxide (H2O2) in the presence of a catalyst such as acetic acid. The reaction is carried out under controlled conditions to ensure the selective oxidation of the nitrogen atom without affecting the ethynyl group.
Industrial Production Methods
In an industrial setting, the production of this compound can be scaled up by optimizing the reaction conditions and using continuous flow reactors. This allows for better control over the reaction parameters, leading to higher yields and purity of the final product.
Chemical Reactions Analysis
Types of Reactions
3-Ethynyl-pyridine 1-oxide undergoes various types of chemical reactions, including:
Oxidation: Further oxidation can lead to the formation of more complex N-oxide derivatives.
Reduction: Reduction reactions can convert the N-oxide back to the parent pyridine compound.
Substitution: The ethynyl group can participate in substitution reactions, leading to the formation of various substituted pyridine derivatives.
Common Reagents and Conditions
Oxidation: Hydrogen peroxide (H2O2) in the presence of acetic acid.
Reduction: Catalytic hydrogenation using palladium on carbon (Pd/C) as a catalyst.
Substitution: Halogenation using reagents such as bromine (Br2) or chlorine (Cl2).
Major Products Formed
Oxidation: Formation of higher N-oxide derivatives.
Reduction: Conversion to 3-Ethynyl-pyridine.
Substitution: Formation of halogenated pyridine derivatives.
Scientific Research Applications
3-Ethynyl-pyridine 1-oxide has several applications in scientific research:
Chemistry: Used as a building block for the synthesis of more complex organic molecules.
Biology: Investigated for its potential biological activities, including antimicrobial and anticancer properties.
Medicine: Explored as a potential drug candidate due to its unique chemical structure.
Industry: Utilized in the production of specialty chemicals and materials.
Mechanism of Action
The mechanism of action of 3-Ethynyl-pyridine 1-oxide involves its interaction with various molecular targets and pathways. The N-oxide group can participate in redox reactions, influencing the oxidative stress within cells. Additionally, the ethynyl group can interact with enzymes and proteins, potentially leading to the modulation of biological pathways.
Comparison with Similar Compounds
Similar Compounds
- 2-Ethynyl-pyridine
- 4-Ethynyl-pyridine
- 3-Ethynyl-thiophene
- 2-Ethynyl-pyrimidine
Uniqueness
3-Ethynyl-pyridine 1-oxide is unique due to the presence of both the ethynyl group and the N-oxide functionality. This combination imparts distinct chemical reactivity and biological activity, making it a valuable compound for various applications.
Properties
IUPAC Name |
3-ethynyl-1-oxidopyridin-1-ium | |
---|---|---|
Details | Computed by LexiChem 2.6.6 (PubChem release 2019.06.18) | |
Source | PubChem | |
URL | https://pubchem.ncbi.nlm.nih.gov | |
Description | Data deposited in or computed by PubChem | |
InChI |
InChI=1S/C7H5NO/c1-2-7-4-3-5-8(9)6-7/h1,3-6H | |
Details | Computed by InChI 1.0.5 (PubChem release 2019.06.18) | |
Source | PubChem | |
URL | https://pubchem.ncbi.nlm.nih.gov | |
Description | Data deposited in or computed by PubChem | |
InChI Key |
VEORPOJZRSZZOX-UHFFFAOYSA-N | |
Details | Computed by InChI 1.0.5 (PubChem release 2019.06.18) | |
Source | PubChem | |
URL | https://pubchem.ncbi.nlm.nih.gov | |
Description | Data deposited in or computed by PubChem | |
Canonical SMILES |
C#CC1=C[N+](=CC=C1)[O-] | |
Details | Computed by OEChem 2.1.5 (PubChem release 2019.06.18) | |
Source | PubChem | |
URL | https://pubchem.ncbi.nlm.nih.gov | |
Description | Data deposited in or computed by PubChem | |
Molecular Formula |
C7H5NO | |
Details | Computed by PubChem 2.1 (PubChem release 2019.06.18) | |
Source | PubChem | |
URL | https://pubchem.ncbi.nlm.nih.gov | |
Description | Data deposited in or computed by PubChem | |
DSSTOX Substance ID |
DTXSID30719390 | |
Record name | 3-Ethynyl-1-oxo-1lambda~5~-pyridine | |
Source | EPA DSSTox | |
URL | https://comptox.epa.gov/dashboard/DTXSID30719390 | |
Description | DSSTox provides a high quality public chemistry resource for supporting improved predictive toxicology. | |
Molecular Weight |
119.12 g/mol | |
Details | Computed by PubChem 2.1 (PubChem release 2021.05.07) | |
Source | PubChem | |
URL | https://pubchem.ncbi.nlm.nih.gov | |
Description | Data deposited in or computed by PubChem | |
CAS No. |
49836-11-5 | |
Record name | 3-Ethynyl-1-oxo-1lambda~5~-pyridine | |
Source | EPA DSSTox | |
URL | https://comptox.epa.gov/dashboard/DTXSID30719390 | |
Description | DSSTox provides a high quality public chemistry resource for supporting improved predictive toxicology. | |
Synthesis routes and methods
Procedure details
Retrosynthesis Analysis
AI-Powered Synthesis Planning: Our tool employs the Template_relevance Pistachio, Template_relevance Bkms_metabolic, Template_relevance Pistachio_ringbreaker, Template_relevance Reaxys, Template_relevance Reaxys_biocatalysis model, leveraging a vast database of chemical reactions to predict feasible synthetic routes.
One-Step Synthesis Focus: Specifically designed for one-step synthesis, it provides concise and direct routes for your target compounds, streamlining the synthesis process.
Accurate Predictions: Utilizing the extensive PISTACHIO, BKMS_METABOLIC, PISTACHIO_RINGBREAKER, REAXYS, REAXYS_BIOCATALYSIS database, our tool offers high-accuracy predictions, reflecting the latest in chemical research and data.
Strategy Settings
Precursor scoring | Relevance Heuristic |
---|---|
Min. plausibility | 0.01 |
Model | Template_relevance |
Template Set | Pistachio/Bkms_metabolic/Pistachio_ringbreaker/Reaxys/Reaxys_biocatalysis |
Top-N result to add to graph | 6 |
Feasible Synthetic Routes
Disclaimer and Information on In-Vitro Research Products
Please be aware that all articles and product information presented on BenchChem are intended solely for informational purposes. The products available for purchase on BenchChem are specifically designed for in-vitro studies, which are conducted outside of living organisms. In-vitro studies, derived from the Latin term "in glass," involve experiments performed in controlled laboratory settings using cells or tissues. It is important to note that these products are not categorized as medicines or drugs, and they have not received approval from the FDA for the prevention, treatment, or cure of any medical condition, ailment, or disease. We must emphasize that any form of bodily introduction of these products into humans or animals is strictly prohibited by law. It is essential to adhere to these guidelines to ensure compliance with legal and ethical standards in research and experimentation.