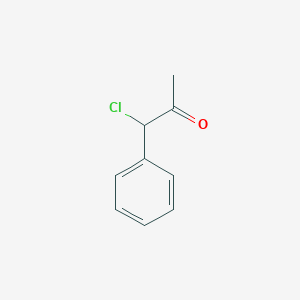
1-Chloro-1-phenyl-propan-2-one
Overview
Description
1-Chloro-1-phenyl-propan-2-one is an organic compound with the molecular formula C9H9ClO It is a chlorinated derivative of phenylacetone and is characterized by the presence of a phenyl group attached to a chlorinated propanone structure
Mechanism of Action
Target of Action
1-Chloro-1-phenyl-propan-2-one is a complex organic compound that has been used in the synthesis of various molecules, including antidepressants . The primary targets of this compound are likely to be the enzymes and receptors involved in the synthesis and action of these antidepressants.
Mode of Action
It is known to be involved in the synthesis of antidepressant molecules via metal-catalyzed reactions . This suggests that it may interact with its targets to facilitate the formation of key structural motifs in these antidepressants.
Biochemical Pathways
The biochemical pathways affected by this compound are likely to be those involved in the synthesis and action of antidepressants. This compound may play a role in the synthesis of key structural motifs in antidepressants such as tricyclic antidepressants (TCAs), selective serotonin reuptake inhibitors (SSRIs), and others . The downstream effects of these pathways could include the alleviation of symptoms and enhancement of the quality of life for individuals with moderate to severe depression .
Result of Action
The molecular and cellular effects of this compound’s action are likely to be related to its role in the synthesis of antidepressants. As part of these reactions, it may contribute to the formation of key structural motifs in these drugs, potentially leading to improved therapeutic outcomes for individuals with depression .
Preparation Methods
1-Chloro-1-phenyl-propan-2-one can be synthesized through several methods. One common laboratory synthesis involves the Friedel-Crafts alkylation reaction of chloroacetone with benzene in the presence of an aluminum chloride catalyst . This reaction proceeds under controlled conditions to yield the desired product. Industrial production methods may involve similar routes but are optimized for larger scale production and efficiency.
Chemical Reactions Analysis
1-Chloro-1-phenyl-propan-2-one undergoes various chemical reactions, including:
Substitution Reactions: The chlorine atom can be substituted by other nucleophiles, such as hydroxide ions, leading to the formation of 1-phenyl-2-propanone.
Reduction Reactions: The compound can be reduced to 1-phenyl-2-propanol using reducing agents like lithium aluminum hydride.
Oxidation Reactions: Oxidation can convert the compound into corresponding carboxylic acids or other oxidized derivatives under specific conditions.
Common reagents used in these reactions include aluminum chloride for substitution, lithium aluminum hydride for reduction, and various oxidizing agents for oxidation. The major products formed depend on the specific reaction conditions and reagents used.
Scientific Research Applications
1-Chloro-1-phenyl-propan-2-one is utilized in several scientific research applications:
Chemistry: It serves as an intermediate in the synthesis of various organic compounds, including pharmaceuticals and agrochemicals.
Biology: The compound is used in biochemical studies to investigate enzyme interactions and metabolic pathways.
Medicine: It is a precursor in the synthesis of certain medicinal compounds, contributing to drug development and research.
Comparison with Similar Compounds
1-Chloro-1-phenyl-propan-2-one can be compared with similar compounds such as:
Phenylacetone: A non-chlorinated analog with similar reactivity but different applications.
1-Phenyl-2-propanone: Another related compound used in similar synthetic applications.
Chloroacetone: A simpler chlorinated ketone used in various chemical reactions.
The uniqueness of this compound lies in its specific reactivity due to the presence of both a phenyl group and a chlorine atom, which influences its chemical behavior and applications .
Properties
IUPAC Name |
1-chloro-1-phenylpropan-2-one | |
---|---|---|
Details | Computed by LexiChem 2.6.6 (PubChem release 2019.06.18) | |
Source | PubChem | |
URL | https://pubchem.ncbi.nlm.nih.gov | |
Description | Data deposited in or computed by PubChem | |
InChI |
InChI=1S/C9H9ClO/c1-7(11)9(10)8-5-3-2-4-6-8/h2-6,9H,1H3 | |
Details | Computed by InChI 1.0.5 (PubChem release 2019.06.18) | |
Source | PubChem | |
URL | https://pubchem.ncbi.nlm.nih.gov | |
Description | Data deposited in or computed by PubChem | |
InChI Key |
KFPLPFVPPOJDFF-UHFFFAOYSA-N | |
Details | Computed by InChI 1.0.5 (PubChem release 2019.06.18) | |
Source | PubChem | |
URL | https://pubchem.ncbi.nlm.nih.gov | |
Description | Data deposited in or computed by PubChem | |
Canonical SMILES |
CC(=O)C(C1=CC=CC=C1)Cl | |
Details | Computed by OEChem 2.1.5 (PubChem release 2019.06.18) | |
Source | PubChem | |
URL | https://pubchem.ncbi.nlm.nih.gov | |
Description | Data deposited in or computed by PubChem | |
Molecular Formula |
C9H9ClO | |
Details | Computed by PubChem 2.1 (PubChem release 2019.06.18) | |
Source | PubChem | |
URL | https://pubchem.ncbi.nlm.nih.gov | |
Description | Data deposited in or computed by PubChem | |
Molecular Weight |
168.62 g/mol | |
Details | Computed by PubChem 2.1 (PubChem release 2021.05.07) | |
Source | PubChem | |
URL | https://pubchem.ncbi.nlm.nih.gov | |
Description | Data deposited in or computed by PubChem | |
CAS No. |
4773-35-7 | |
Record name | NSC38444 | |
Source | DTP/NCI | |
URL | https://dtp.cancer.gov/dtpstandard/servlet/dwindex?searchtype=NSC&outputformat=html&searchlist=38444 | |
Description | The NCI Development Therapeutics Program (DTP) provides services and resources to the academic and private-sector research communities worldwide to facilitate the discovery and development of new cancer therapeutic agents. | |
Explanation | Unless otherwise indicated, all text within NCI products is free of copyright and may be reused without our permission. Credit the National Cancer Institute as the source. | |
Retrosynthesis Analysis
AI-Powered Synthesis Planning: Our tool employs the Template_relevance Pistachio, Template_relevance Bkms_metabolic, Template_relevance Pistachio_ringbreaker, Template_relevance Reaxys, Template_relevance Reaxys_biocatalysis model, leveraging a vast database of chemical reactions to predict feasible synthetic routes.
One-Step Synthesis Focus: Specifically designed for one-step synthesis, it provides concise and direct routes for your target compounds, streamlining the synthesis process.
Accurate Predictions: Utilizing the extensive PISTACHIO, BKMS_METABOLIC, PISTACHIO_RINGBREAKER, REAXYS, REAXYS_BIOCATALYSIS database, our tool offers high-accuracy predictions, reflecting the latest in chemical research and data.
Strategy Settings
Precursor scoring | Relevance Heuristic |
---|---|
Min. plausibility | 0.01 |
Model | Template_relevance |
Template Set | Pistachio/Bkms_metabolic/Pistachio_ringbreaker/Reaxys/Reaxys_biocatalysis |
Top-N result to add to graph | 6 |
Feasible Synthetic Routes
Disclaimer and Information on In-Vitro Research Products
Please be aware that all articles and product information presented on BenchChem are intended solely for informational purposes. The products available for purchase on BenchChem are specifically designed for in-vitro studies, which are conducted outside of living organisms. In-vitro studies, derived from the Latin term "in glass," involve experiments performed in controlled laboratory settings using cells or tissues. It is important to note that these products are not categorized as medicines or drugs, and they have not received approval from the FDA for the prevention, treatment, or cure of any medical condition, ailment, or disease. We must emphasize that any form of bodily introduction of these products into humans or animals is strictly prohibited by law. It is essential to adhere to these guidelines to ensure compliance with legal and ethical standards in research and experimentation.