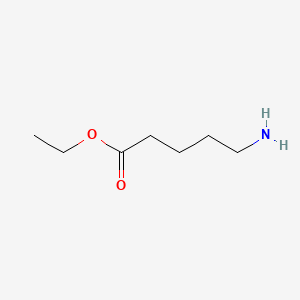
Ethyl 5-aminovalerate
Overview
Description
Ethyl 5-aminovalerate (CAS: 29840-57-1) is an ester derivative of 5-aminovalerate (5AVA), a five-carbon ω-amino acid. Its molecular formula is C₇H₁₅NO₂·ClH, with a molecular weight of 181.7 g/mol . This compound is primarily characterized in safety documentation, highlighting hazards such as skin/eye irritation and toxicity upon ingestion or inhalation . Its industrial applications are inferred from its structural relationship to 5AVA, which is widely used in bioplastics (e.g., nylon 5,5) and disposable goods .
Biological Activity
Ethyl 5-aminovalerate (E5AV) is an organic compound with significant biological activities, primarily due to its structural similarity to amino acids. This compound is a derivative of 5-aminovaleric acid and functions as a precursor in various biochemical pathways. Its potential applications span pharmaceuticals, biochemistry, and bioplastics production. This article explores the biological activities of E5AV, including its synthesis, metabolic pathways, and case studies highlighting its therapeutic potential.
- Molecular Formula : C₅H₁₁N O₂
- Molar Mass : Approximately 145.20 g/mol
- Structure : E5AV features an ethyl ester functional group attached to a five-carbon chain, which influences its biological activity and solubility in aqueous environments.
1. Anticancer Properties
E5AV has shown promising anticancer activity in various studies. A derivative of E5AV demonstrated significant inhibition of tumor cell colony formation and migration in HeLa and HT-29 cancer cell lines. The IC50 values were reported as 0.737 ± 0.05 μM for HeLa cells and 1.194 ± 0.02 μM for HT-29 cells, indicating potent cytotoxic effects . The mechanism involves the induction of early apoptosis and disruption of mitochondrial membrane potential, suggesting that E5AV derivatives could serve as lead compounds for cancer drug development.
2. Metabolic Pathways
E5AV is involved in metabolic pathways as a metabolite of L-lysine catabolism. It can be synthesized through enzymatic reactions involving L-lysine monooxygenase (DavB) and 5-aminovaleramide amidohydrolase (DavA), which convert L-lysine into E5AV through a two-enzyme coupled system . This pathway not only highlights the metabolic significance of E5AV but also its potential as a C5 platform chemical for producing various bioplastics.
3. Enzyme Inhibition
Research has indicated that E5AV can act as an inhibitor for certain enzymes, such as trypsin. It has been observed that amino acid esters like hexyl β-amino valerate inhibit the hydrolysis of specific substrates by trypsin, suggesting potential applications in modulating enzyme activity .
Synthesis Methods
E5AV can be synthesized through several methods:
- Biotransformation : Utilizing engineered Escherichia coli strains to convert L-lysine into E5AV via enzymatic pathways.
- Chemical Synthesis : Various chemical routes exist for synthesizing E5AV from simpler precursors, allowing for tailored modifications depending on desired applications .
Case Study 1: Anticancer Activity
A study focused on the anticancer properties of E5AV derivatives reported significant antiadhesive effects on HeLa cells and antitubulin activity, leading to early apoptosis induction. This study emphasizes the compound's potential in developing novel anticancer therapies .
Case Study 2: Bioplastic Production
Research has explored the production of E5AV from renewable feedstocks as a sustainable method for synthesizing bioplastics like nylon 5. The engineered biosynthetic pathways have achieved high yields of E5AV, demonstrating its viability as an industrially relevant compound .
Comparative Analysis
The following table summarizes the unique features and biological activities of related compounds:
Compound | Structure | Biological Activity |
---|---|---|
Ethyl 4-aminobutyrate | C₆H₁₃NO₂ | Involved in GABA metabolism |
Ethyl 6-aminohexanoate | C₈H₁₉NO₂ | Different metabolic pathways |
Ethyl 3-amino-propanoate | C₅H₁₃NO₂ | More polar due to proximity of amine |
This compound | C₅H₁₁N O₂ | Anticancer activity, metabolic precursor |
Q & A
Basic Research Questions
Q. What are the established enzymatic pathways for synthesizing ethyl 5-aminovalerate from L-lysine in microbial systems?
Two primary pathways are documented:
- DavB/DavA Pathway : L-lysine is oxidized to 5-aminovaleramide by lysine 2-monooxygenase (DavB), followed by hydrolysis to 5-aminovalerate via 5-aminovaleramide amidohydrolase (DavA). This pathway, derived from Pseudomonas spp., achieves yields of ~20.8 g/L 5-aminovalerate from 30 g/L L-lysine in 12 hours using purified enzymes .
- L-Lysine α-Oxidase Pathway : Oxidation of L-lysine by Trichoderma viride α-oxidase generates 6-amino-2-oxocaproic acid, which undergoes spontaneous decarboxylation to 5-aminovalerate. However, this method is less efficient (13.4 g/L from 17.5 g/L L-lysine over 5 days) . Methodological Note: Validate pathway efficiency using HPLC or LC-MS to quantify intermediates and final products, and compare kinetic parameters (e.g., , ) of recombinant enzymes .
Q. How can researchers optimize experimental conditions for whole-cell biocatalysis of this compound production?
Key factors include:
- Substrate Concentration : Maintain L-lysine concentrations below inhibitory thresholds (e.g., <30 g/L) to prevent feedback inhibition .
- Strain Engineering : Overexpress transporter proteins (e.g., lysP in E. coli) to enhance substrate uptake and reduce intermediate accumulation .
- Induction Timing : Use inducible promoters (e.g., nirB under anaerobic conditions) to synchronize enzyme expression with metabolic activity . Data-Driven Tip: Conduct fractional factorial designs to identify optimal pH (6.5–7.5), temperature (30–37°C), and aeration conditions .
Advanced Research Questions
Q. What computational and experimental approaches resolve bottlenecks in artificial biosynthetic pathways for this compound?
- Computational Tools : Use ReactPRED and MINEs databases to predict feasible enzymatic routes and intermediate toxicity . For example, ReactPRED proposed levulinic acid pathways, though validation remains pending .
- Parameter Estimation : Apply Markov Chain Monte Carlo (MCMC) methods to fit normalized kinetic models to multi-omics data, enabling precise tuning of metabolite fluxes .
- CRISPR Interference : Knock down competing pathways (e.g., GABA shunt) to redirect carbon flux toward 5-aminovalerate . Validation Step: Couple proteomics (e.g., SILAC) with C metabolic flux analysis to quantify pathway activity .
Q. How can conflicting data on intermediate accumulation (e.g., 5-aminovalerate vs. glutaric acid) be systematically analyzed?
- Metabolic Engineering : Engineer Corynebacterium glutamicum to overexpress importer proteins (e.g., ydcE), which reduces extracellular 5-aminovalerate accumulation by 90%, favoring glutaric acid production .
- Statistical Analysis : Perform ANOVA or t-tests on triplicate bioreactor runs to assess significance of pathway modifications. For example, Wittmann et al. reported a 2.5-fold increase in glutaric acid titers after transporter overexpression .
- Multi-Omics Integration : Combine RNA-seq and metabolomics to identify regulatory nodes (e.g., NADH/NAD+ ratios) that imbalance intermediate turnover .
Q. What methodologies ensure reproducibility in strain performance across different laboratories?
- Standardized Protocols : Document strain lineage (e.g., E. coli BL21(DE3) pET-28a(+)-davAB), growth media (e.g., M9 + 2% glucose), and induction conditions (e.g., 0.1 mM IPTG) .
- Data Transparency : Publish raw metabolomics data in repositories like MetaboLights, including error bars for biological replicates (n ≥ 3) .
- Interlab Studies : Collaborate with third-party labs to validate titers using harmonized analytical methods (e.g., GC-FID for glutaric acid quantification) .
Comparison with Similar Compounds
Production Methods and Yields
5-Aminovalerate (5AVA)
- Microbial Production: Engineered Escherichia coli and Corynebacterium glutamicum are primary hosts. E. coli: Achieves 50.62 g/L 5AVA from 100 g/L L-lysine HCl via ethanol-pretreated whole-cell catalysis (yield: 0.84 mol/mol) . Overexpression of transport proteins (LysP, PP2911) increases yields to 0.94 mol/mol . Fed-batch processes yield 63.2 g/L . C. glutamicum: Fed-batch fermentation produces 28 g/L 5AVA and 7 g/L glutarate, with superior yields (275 mmol/mol glucose) compared to E. coli .
- Key Factors: Ethanol pretreatment reduces H₂O₂ toxicity ; pH 7.0 optimizes enzymatic conversion .
δ-Valerolactam
- Coproduct with 5AVA : Synthesized via pH-dependent equilibrium in E. coli.
- Applications : Precursor for nylon 5, synthesized with ε-caprolactam .
Glutarate
- Byproduct in C. glutamicum: Produced via endogenous transamination of 5AVA. Glutarate yields reach 123 mmol/mol glucose, exceeding 5AVA yields in C. glutamicum . E. coli requires heterologous davDT genes for glutarate synthesis, achieving 68 mmol/mol .
Key Differences and Advantages
Metabolic Pathways: E. coli lacks endogenous 5AVA re-uptake, enabling high extracellular accumulation . C. glutamicum naturally secretes glutarate, making it superior for mixed-product processes .
pH Sensitivity :
- δ-Valerolactam production is favored under alkaline conditions (pH 9.0), while 5AVA dominates at pH 5.0–7.0 .
Yield Efficiency :
Data Tables
Table 1: Production Metrics of 5AVA and Related Compounds
Properties
IUPAC Name |
ethyl 5-aminopentanoate | |
---|---|---|
Details | Computed by Lexichem TK 2.7.0 (PubChem release 2021.05.07) | |
Source | PubChem | |
URL | https://pubchem.ncbi.nlm.nih.gov | |
Description | Data deposited in or computed by PubChem | |
InChI |
InChI=1S/C7H15NO2/c1-2-10-7(9)5-3-4-6-8/h2-6,8H2,1H3 | |
Details | Computed by InChI 1.0.6 (PubChem release 2021.05.07) | |
Source | PubChem | |
URL | https://pubchem.ncbi.nlm.nih.gov | |
Description | Data deposited in or computed by PubChem | |
InChI Key |
YMYLIDVSLIIAPI-UHFFFAOYSA-N | |
Details | Computed by InChI 1.0.6 (PubChem release 2021.05.07) | |
Source | PubChem | |
URL | https://pubchem.ncbi.nlm.nih.gov | |
Description | Data deposited in or computed by PubChem | |
Canonical SMILES |
CCOC(=O)CCCCN | |
Details | Computed by OEChem 2.3.0 (PubChem release 2021.05.07) | |
Source | PubChem | |
URL | https://pubchem.ncbi.nlm.nih.gov | |
Description | Data deposited in or computed by PubChem | |
Molecular Formula |
C7H15NO2 | |
Details | Computed by PubChem 2.1 (PubChem release 2021.05.07) | |
Source | PubChem | |
URL | https://pubchem.ncbi.nlm.nih.gov | |
Description | Data deposited in or computed by PubChem | |
DSSTOX Substance ID |
DTXSID30466791 | |
Record name | ETHYL 5-AMINOVALERATE | |
Source | EPA DSSTox | |
URL | https://comptox.epa.gov/dashboard/DTXSID30466791 | |
Description | DSSTox provides a high quality public chemistry resource for supporting improved predictive toxicology. | |
Molecular Weight |
145.20 g/mol | |
Details | Computed by PubChem 2.1 (PubChem release 2021.05.07) | |
Source | PubChem | |
URL | https://pubchem.ncbi.nlm.nih.gov | |
Description | Data deposited in or computed by PubChem | |
CAS No. |
26342-05-2 | |
Record name | ETHYL 5-AMINOVALERATE | |
Source | EPA DSSTox | |
URL | https://comptox.epa.gov/dashboard/DTXSID30466791 | |
Description | DSSTox provides a high quality public chemistry resource for supporting improved predictive toxicology. | |
Retrosynthesis Analysis
AI-Powered Synthesis Planning: Our tool employs the Template_relevance Pistachio, Template_relevance Bkms_metabolic, Template_relevance Pistachio_ringbreaker, Template_relevance Reaxys, Template_relevance Reaxys_biocatalysis model, leveraging a vast database of chemical reactions to predict feasible synthetic routes.
One-Step Synthesis Focus: Specifically designed for one-step synthesis, it provides concise and direct routes for your target compounds, streamlining the synthesis process.
Accurate Predictions: Utilizing the extensive PISTACHIO, BKMS_METABOLIC, PISTACHIO_RINGBREAKER, REAXYS, REAXYS_BIOCATALYSIS database, our tool offers high-accuracy predictions, reflecting the latest in chemical research and data.
Strategy Settings
Precursor scoring | Relevance Heuristic |
---|---|
Min. plausibility | 0.01 |
Model | Template_relevance |
Template Set | Pistachio/Bkms_metabolic/Pistachio_ringbreaker/Reaxys/Reaxys_biocatalysis |
Top-N result to add to graph | 6 |
Feasible Synthetic Routes
Disclaimer and Information on In-Vitro Research Products
Please be aware that all articles and product information presented on BenchChem are intended solely for informational purposes. The products available for purchase on BenchChem are specifically designed for in-vitro studies, which are conducted outside of living organisms. In-vitro studies, derived from the Latin term "in glass," involve experiments performed in controlled laboratory settings using cells or tissues. It is important to note that these products are not categorized as medicines or drugs, and they have not received approval from the FDA for the prevention, treatment, or cure of any medical condition, ailment, or disease. We must emphasize that any form of bodily introduction of these products into humans or animals is strictly prohibited by law. It is essential to adhere to these guidelines to ensure compliance with legal and ethical standards in research and experimentation.