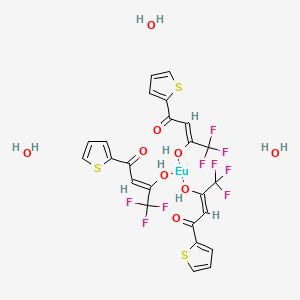
Europium (III) thenoyltrifluoroacetonate trihydrate
Overview
Description
Europium (III) thenoyltrifluoroacetonate trihydrate is an organometallic compound with the chemical formula C24H12EuF9O6S3·3H2O. It is known for its luminescent properties and is used in various scientific and industrial applications. The compound consists of europium ions coordinated with thenoyltrifluoroacetonate ligands and water molecules.
Mechanism of Action
Target of Action
Europium (III) thenoyltrifluoroacetonate trihydrate is primarily used as a luminescent laser dye . The primary targets of this compound are therefore the light-sensitive molecules and structures within the application environment. The compound’s luminescent properties make it particularly useful in applications that require the emission of light, such as in lasers and other optical devices.
Mode of Action
The mode of action of this compound is based on its luminescent properties. When excited by an external energy source, such as a laser, the compound emits light. This is due to the unique electronic structure of the europium ion, which allows it to absorb energy and then release it in the form of light. The exact wavelength of the emitted light can be tuned by adjusting the surrounding chemical environment, making this compound highly versatile in optical applications .
Biochemical Pathways
As a luminescent compound, this compound does not directly interact with biochemical pathways within a biological organism. Instead, its primary interactions are with light and energy, as described above. It’s worth noting that the compound’s structure is achieved by the formation of hydrogen bonds of a water molecule with oxygen atoms of two neighboring thenoyltrifluoroacetonate ions .
Pharmacokinetics
It is also likely to have low bioavailability due to its poor solubility in water .
Result of Action
The primary result of the action of this compound is the emission of light. This makes it a valuable tool in various technological applications, particularly those involving lasers and other optical devices .
Biochemical Analysis
Biochemical Properties
The nature of these interactions is complex and may vary depending on the specific biochemical context .
Cellular Effects
It has been used as a fluorescent thermosensor in intracellular thermometry . This suggests that it may influence cell function, potentially impacting cell signaling pathways, gene expression, and cellular metabolism .
Molecular Mechanism
It’s known that the fluorescence intensity of the compound decreases in response to an increase in temperature, suggesting a potential role in thermal biology .
Preparation Methods
Synthetic Routes and Reaction Conditions
The synthesis of europium (III) thenoyltrifluoroacetonate trihydrate typically involves the reaction of europium chloride with thenoyltrifluoroacetone in the presence of a base, such as sodium hydroxide. The reaction is carried out in an organic solvent, such as ethanol, under reflux conditions. The product is then purified by recrystallization from an appropriate solvent .
Industrial Production Methods
Industrial production methods for this compound are similar to laboratory synthesis but are scaled up to accommodate larger quantities. The process involves the same reactants and conditions but utilizes larger reaction vessels and more efficient purification techniques to ensure high yield and purity .
Chemical Reactions Analysis
Types of Reactions
Europium (III) thenoyltrifluoroacetonate trihydrate undergoes various chemical reactions, including:
Oxidation: The compound can be oxidized under specific conditions, leading to the formation of europium (IV) complexes.
Reduction: Reduction reactions can convert europium (III) to europium (II) complexes.
Substitution: Ligand substitution reactions can occur, where the thenoyltrifluoroacetonate ligands are replaced by other ligands.
Common Reagents and Conditions
Common reagents used in these reactions include oxidizing agents like hydrogen peroxide for oxidation, reducing agents like sodium borohydride for reduction, and various ligands for substitution reactions. The reactions are typically carried out under controlled temperature and pH conditions to ensure the desired products are formed .
Major Products
The major products formed from these reactions include europium (IV) and europium (II) complexes, as well as substituted europium (III) complexes with different ligands .
Scientific Research Applications
Europium (III) thenoyltrifluoroacetonate trihydrate has a wide range of scientific research applications, including:
Chemistry: Used as a luminescent probe in various chemical analyses and as a catalyst in organic synthesis.
Biology: Employed in intracellular thermometry to measure temperature variations within cells.
Industry: Utilized in the manufacturing of light-emitting diodes (LEDs) and other luminescent materials.
Comparison with Similar Compounds
Similar Compounds
Europium (III) tetrakis-thenoyltrifluoroacetonate: Another luminescent europium complex with similar properties.
Europium (III) acetylacetonate: A europium complex with acetylacetonate ligands, used in similar applications.
Europium (III) dibenzoylmethane: A europium complex with dibenzoylmethane ligands, also known for its luminescent properties.
Uniqueness
Europium (III) thenoyltrifluoroacetonate trihydrate is unique due to its specific ligand structure, which provides distinct luminescent properties and makes it particularly useful in applications requiring high sensitivity and specificity .
Properties
IUPAC Name |
europium;(Z)-4,4,4-trifluoro-3-hydroxy-1-thiophen-2-ylbut-2-en-1-one;trihydrate | |
---|---|---|
Details | Computed by Lexichem TK 2.7.0 (PubChem release 2021.05.07) | |
Source | PubChem | |
URL | https://pubchem.ncbi.nlm.nih.gov | |
Description | Data deposited in or computed by PubChem | |
InChI |
InChI=1S/3C8H5F3O2S.Eu.3H2O/c3*9-8(10,11)7(13)4-5(12)6-2-1-3-14-6;;;;/h3*1-4,13H;;3*1H2/b3*7-4-;;;; | |
Details | Computed by InChI 1.0.6 (PubChem release 2021.05.07) | |
Source | PubChem | |
URL | https://pubchem.ncbi.nlm.nih.gov | |
Description | Data deposited in or computed by PubChem | |
InChI Key |
KCPUGEHVYLTNMO-CIHVUJSSSA-N | |
Details | Computed by InChI 1.0.6 (PubChem release 2021.05.07) | |
Source | PubChem | |
URL | https://pubchem.ncbi.nlm.nih.gov | |
Description | Data deposited in or computed by PubChem | |
Canonical SMILES |
C1=CSC(=C1)C(=O)C=C(C(F)(F)F)O.C1=CSC(=C1)C(=O)C=C(C(F)(F)F)O.C1=CSC(=C1)C(=O)C=C(C(F)(F)F)O.O.O.O.[Eu] | |
Details | Computed by OEChem 2.3.0 (PubChem release 2021.05.07) | |
Source | PubChem | |
URL | https://pubchem.ncbi.nlm.nih.gov | |
Description | Data deposited in or computed by PubChem | |
Isomeric SMILES |
C1=CSC(=C1)C(=O)/C=C(\O)/C(F)(F)F.C1=CSC(=C1)C(=O)/C=C(\O)/C(F)(F)F.C1=CSC(=C1)C(=O)/C=C(\O)/C(F)(F)F.O.O.O.[Eu] | |
Details | Computed by OEChem 2.3.0 (PubChem release 2021.05.07) | |
Source | PubChem | |
URL | https://pubchem.ncbi.nlm.nih.gov | |
Description | Data deposited in or computed by PubChem | |
Molecular Formula |
C24H21EuF9O9S3 | |
Details | Computed by PubChem 2.1 (PubChem release 2021.05.07) | |
Source | PubChem | |
URL | https://pubchem.ncbi.nlm.nih.gov | |
Description | Data deposited in or computed by PubChem | |
Molecular Weight |
872.6 g/mol | |
Details | Computed by PubChem 2.1 (PubChem release 2021.05.07) | |
Source | PubChem | |
URL | https://pubchem.ncbi.nlm.nih.gov | |
Description | Data deposited in or computed by PubChem | |
Retrosynthesis Analysis
AI-Powered Synthesis Planning: Our tool employs the Template_relevance Pistachio, Template_relevance Bkms_metabolic, Template_relevance Pistachio_ringbreaker, Template_relevance Reaxys, Template_relevance Reaxys_biocatalysis model, leveraging a vast database of chemical reactions to predict feasible synthetic routes.
One-Step Synthesis Focus: Specifically designed for one-step synthesis, it provides concise and direct routes for your target compounds, streamlining the synthesis process.
Accurate Predictions: Utilizing the extensive PISTACHIO, BKMS_METABOLIC, PISTACHIO_RINGBREAKER, REAXYS, REAXYS_BIOCATALYSIS database, our tool offers high-accuracy predictions, reflecting the latest in chemical research and data.
Strategy Settings
Precursor scoring | Relevance Heuristic |
---|---|
Min. plausibility | 0.01 |
Model | Template_relevance |
Template Set | Pistachio/Bkms_metabolic/Pistachio_ringbreaker/Reaxys/Reaxys_biocatalysis |
Top-N result to add to graph | 6 |
Feasible Synthetic Routes
Disclaimer and Information on In-Vitro Research Products
Please be aware that all articles and product information presented on BenchChem are intended solely for informational purposes. The products available for purchase on BenchChem are specifically designed for in-vitro studies, which are conducted outside of living organisms. In-vitro studies, derived from the Latin term "in glass," involve experiments performed in controlled laboratory settings using cells or tissues. It is important to note that these products are not categorized as medicines or drugs, and they have not received approval from the FDA for the prevention, treatment, or cure of any medical condition, ailment, or disease. We must emphasize that any form of bodily introduction of these products into humans or animals is strictly prohibited by law. It is essential to adhere to these guidelines to ensure compliance with legal and ethical standards in research and experimentation.