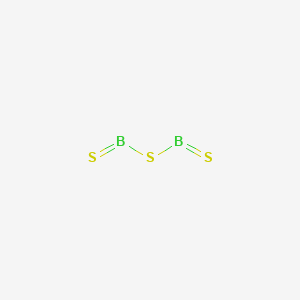
Boron sulfide
Overview
Description
Boron sulfide, with the chemical formula ( \text{B}_2\text{S}_3 ), is a white, moisture-sensitive solid. It is known for its polymeric structure and has been of interest in various high-tech applications, including the production of specialized glasses and as a reagent in organic synthesis .
Synthetic Routes and Reaction Conditions:
Direct Synthesis: The earliest method of synthesizing this compound involved the direct reaction of amorphous boron with sulfur vapor. This method was first reported by Jöns Jakob Berzelius in 1824: [ 2 \text{B} + 3 \text{S} \rightarrow \text{B}_2\text{S}_3 ]
Hydrogen Sulfide Method: Another synthesis method, favored by Friedrich Wöhler and Henri Etienne Sainte-Claire Deville in 1858, involves the reaction of boron with hydrogen sulfide: [ 2 \text{B} + 3 \text{H}_2\text{S} \rightarrow \text{B}_2\text{S}_3 + 3 \text{H}_2 ]
Iron and Manganese Borides Method: A more modern approach involves reacting iron and manganese borides with hydrogen sulfide at temperatures around 300°C: [ 2 \text{FeB} + 4 \text{H}_2\text{S} \rightarrow \text{B}_2\text{S}_3 + \text{FeS} + 4 \text{H}_2 ]
Industrial Production Methods: Industrial production of this compound typically follows the hydrogen sulfide method due to its efficiency and the availability of raw materials .
Types of Reactions:
Hydrolysis: this compound reacts readily with water, including atmospheric moisture, to produce boric acid and hydrogen sulfide: [ \text{B}_2\text{S}_3 + 3 \text{H}_2\text{O} \rightarrow \text{B}_2\text{O}_3 + 3 \text{H}_2\text{S} ]
Conversion to Thiones: It can convert ketones into their corresponding thiones. For example, benzophenone is converted to its thione: [ \text{B}_2\text{S}_3 + 3 (\text{C}_6\text{H}_5)_2\text{C=O} \rightarrow \text{B}_2\text{O}_3 + 3 (\text{C}_6\text{H}_5)_2\text{C=S} ]
Common Reagents and Conditions:
Water: For hydrolysis reactions.
Ketones: For conversion to thiones, typically under mild conditions.
Major Products:
Boric Acid: From hydrolysis.
Thiones: From reactions with ketones.
Scientific Research Applications
Electrocatalysis
Boron sulfide has been investigated as a catalyst in the electrochemical oxygen evolution reaction (OER). A study demonstrated that ternary pyrite-type this compound, when coated with amorphous FeCoNiB, exhibited superior OER activity with an overpotential of 419.4 mV at 100 mA cm in alkaline conditions. This hybrid catalyst showed excellent stability over extended periods, making it a promising candidate for energy conversion technologies .
Synthesis of Chalcogenide Perovskites
Research has utilized this compound for synthesizing chalcogenide perovskites (CPs) through sulfurization of binary and ternary oxides. This method yielded pure-phase crystalline CPs at temperatures as low as 600 °C with over 95% yields, showcasing the efficiency and safety of using this compound in material synthesis .
Boron Doping to Mitigate Sulfur Poisoning
In catalytic processes, sulfur poisoning poses a significant challenge. Recent studies have shown that boron doping can enhance the resistance of metal catalysts (e.g., Pd, Pt) to sulfur poisoning. Theoretical calculations indicate that boron modifies the electronic properties of these metals, improving their catalytic performance in hydrogen production from HS splitting .
Advanced Ceramics
This compound is employed as an additive in advanced ceramics to enhance durability and thermal resistance. Its incorporation into ceramic composites results in materials capable of withstanding extreme conditions typical in aerospace and automotive applications .
Optical Materials
Due to its infrared transparency and stability, this compound is utilized in specialized optical coatings and glass. These materials are essential for applications in thermal imaging and environmental sensors, where improved light transmission is critical .
Semiconductor Technologies
In semiconductor applications, this compound serves as a dopant or thin-film coating material. Its chemical reactivity allows for modifications of electronic and optical properties essential for developing transistors and sensors .
Case Studies
Mechanism of Action
The primary mechanism by which boron sulfide exerts its effects is through its reactivity with water and organic compounds. The hydrolysis reaction releases hydrogen sulfide, which can further participate in various chemical processes. The conversion of ketones to thiones involves the replacement of the oxygen atom in the carbonyl group with a sulfur atom from this compound .
Comparison with Similar Compounds
Boron Trichloride (BCl3): Like boron sulfide, boron trichloride is used in organic synthesis but is more commonly employed as a catalyst and in the production of high-purity boron.
Lawesson’s Reagent: Another sulfur-containing compound used for the conversion of carbonyl compounds to thiones, similar to this compound but with different reactivity and applications.
Uniqueness: this compound is unique in its ability to form polymeric structures and its specific reactivity with water and organic compounds, making it a valuable reagent in specialized chemical syntheses .
Biological Activity
Boron sulfide (B₂S₃) is a compound that has garnered attention in various fields, including medicinal chemistry and bioinorganic research. This article explores the biological activity of this compound, synthesizing findings from diverse studies to provide a comprehensive overview.
This compound is characterized by its unique structure, consisting of boron and sulfur atoms. It exists in several forms, including amorphous and crystalline states. The compound's molecular structure influences its reactivity and interaction with biological systems.
Biological Activity Overview
Boron-containing compounds, including this compound, have been investigated for their potential biological activities. Notably, boron is recognized as an essential micronutrient in plants and has been shown to stimulate microbial activity in soils, which indicates its importance in ecological systems .
1. Antitumor Activity
Recent studies have highlighted the antitumor potential of boron-containing compounds through Boron Neutron Capture Therapy (BNCT). For instance, research has shown that certain boron-enriched compounds exhibit significant cytotoxicity against cancer cell lines, such as A549 (lung cancer) cells. The intracellular uptake of these compounds is crucial for their effectiveness .
Table 1: Cytotoxic Activity of Boron-Containing Compounds
Compound | Cell Line | Cytotoxicity (IC50 μM) |
---|---|---|
10B-15b | A549 | 34 ± 1 |
10B-16b | A549 | 18 ± 3 |
10B-17a | A549 | 20 ± 1 |
This table summarizes the cytotoxic effects observed in various boron-containing compounds against A549 cells, indicating their potential use in cancer therapy.
2. Sedative Effects
Another area of interest is the sedative properties of certain boron-containing derivatives. Studies involving animal models have demonstrated that specific boron derivatives can induce sedation with varying durations depending on their chemical structure. For example, one compound induced sedation lasting over 48 hours at a dose of 600 mg/kg, while another had a much shorter effect .
Table 2: Sedative Effects of Boron Derivatives
Compound | Dose (mg/kg) | Duration of Sedation |
---|---|---|
FB-1 | 600 | >48 hours |
AB-1 | 600 | <6 hours |
This table illustrates the differences in sedative effects between two boron derivatives.
The biological activity of this compound and its derivatives can be attributed to several mechanisms:
- Interaction with Biomolecules : Boron compounds are known to form strong interactions with biomolecules such as proteins and nucleic acids. This property is essential for their therapeutic effects, particularly in targeting cancer cells .
- Endocytosis : The uptake of boron-containing compounds into cells often occurs through endocytosis, which is influenced by temperature and the presence of inhibitors .
Case Study 1: Boron Neutron Capture Therapy
In a clinical study on BNCT, patients with specific types of tumors were treated using boron-containing compounds followed by neutron irradiation. The results indicated that higher intracellular boron concentrations correlated with improved therapeutic outcomes .
Case Study 2: Sedative Effects in Animal Models
A study evaluated the sedative effects of two distinct boron derivatives on mice. The findings revealed significant differences in onset time and duration of sedation between the compounds, suggesting that structural variations can significantly impact biological activity .
Properties
IUPAC Name |
sulfanylidene(sulfanylideneboranylsulfanyl)borane | |
---|---|---|
Details | Computed by LexiChem 2.6.6 (PubChem release 2019.06.18) | |
Source | PubChem | |
URL | https://pubchem.ncbi.nlm.nih.gov | |
Description | Data deposited in or computed by PubChem | |
InChI |
InChI=1S/B2S3/c3-1-5-2-4 | |
Details | Computed by InChI 1.0.5 (PubChem release 2019.06.18) | |
Source | PubChem | |
URL | https://pubchem.ncbi.nlm.nih.gov | |
Description | Data deposited in or computed by PubChem | |
InChI Key |
ZVTQDOIPKNCMAR-UHFFFAOYSA-N | |
Details | Computed by InChI 1.0.5 (PubChem release 2019.06.18) | |
Source | PubChem | |
URL | https://pubchem.ncbi.nlm.nih.gov | |
Description | Data deposited in or computed by PubChem | |
Canonical SMILES |
B(=S)SB=S | |
Details | Computed by OEChem 2.1.5 (PubChem release 2019.06.18) | |
Source | PubChem | |
URL | https://pubchem.ncbi.nlm.nih.gov | |
Description | Data deposited in or computed by PubChem | |
Molecular Formula |
B2S3 | |
Details | Computed by PubChem 2.1 (PubChem release 2019.06.18) | |
Record name | boron sulfide | |
Source | Wikipedia | |
URL | https://en.wikipedia.org/wiki/Boron_sulfide | |
Description | Chemical information link to Wikipedia. | |
Details | Computed by PubChem 2.1 (PubChem release 2019.06.18) | |
Source | PubChem | |
URL | https://pubchem.ncbi.nlm.nih.gov | |
Description | Data deposited in or computed by PubChem | |
DSSTOX Substance ID |
DTXSID50923210 | |
Record name | Diborathiane-1,3-dithione | |
Source | EPA DSSTox | |
URL | https://comptox.epa.gov/dashboard/DTXSID50923210 | |
Description | DSSTox provides a high quality public chemistry resource for supporting improved predictive toxicology. | |
Molecular Weight |
117.8 g/mol | |
Details | Computed by PubChem 2.1 (PubChem release 2021.05.07) | |
Source | PubChem | |
URL | https://pubchem.ncbi.nlm.nih.gov | |
Description | Data deposited in or computed by PubChem | |
CAS No. |
12007-33-9 | |
Record name | Diboron trisulphide | |
Source | ChemIDplus | |
URL | https://pubchem.ncbi.nlm.nih.gov/substance/?source=chemidplus&sourceid=0012007339 | |
Description | ChemIDplus is a free, web search system that provides access to the structure and nomenclature authority files used for the identification of chemical substances cited in National Library of Medicine (NLM) databases, including the TOXNET system. | |
Record name | Diborathiane-1,3-dithione | |
Source | EPA DSSTox | |
URL | https://comptox.epa.gov/dashboard/DTXSID50923210 | |
Description | DSSTox provides a high quality public chemistry resource for supporting improved predictive toxicology. | |
Record name | Diboron trisulphide | |
Source | European Chemicals Agency (ECHA) | |
URL | https://echa.europa.eu/substance-information/-/substanceinfo/100.031.355 | |
Description | The European Chemicals Agency (ECHA) is an agency of the European Union which is the driving force among regulatory authorities in implementing the EU's groundbreaking chemicals legislation for the benefit of human health and the environment as well as for innovation and competitiveness. | |
Explanation | Use of the information, documents and data from the ECHA website is subject to the terms and conditions of this Legal Notice, and subject to other binding limitations provided for under applicable law, the information, documents and data made available on the ECHA website may be reproduced, distributed and/or used, totally or in part, for non-commercial purposes provided that ECHA is acknowledged as the source: "Source: European Chemicals Agency, http://echa.europa.eu/". Such acknowledgement must be included in each copy of the material. ECHA permits and encourages organisations and individuals to create links to the ECHA website under the following cumulative conditions: Links can only be made to webpages that provide a link to the Legal Notice page. | |
Disclaimer and Information on In-Vitro Research Products
Please be aware that all articles and product information presented on BenchChem are intended solely for informational purposes. The products available for purchase on BenchChem are specifically designed for in-vitro studies, which are conducted outside of living organisms. In-vitro studies, derived from the Latin term "in glass," involve experiments performed in controlled laboratory settings using cells or tissues. It is important to note that these products are not categorized as medicines or drugs, and they have not received approval from the FDA for the prevention, treatment, or cure of any medical condition, ailment, or disease. We must emphasize that any form of bodily introduction of these products into humans or animals is strictly prohibited by law. It is essential to adhere to these guidelines to ensure compliance with legal and ethical standards in research and experimentation.