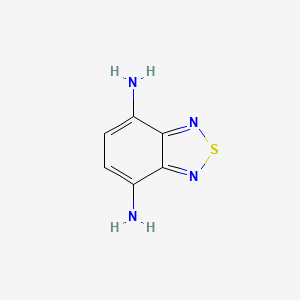
2,1,3-Benzothiadiazole-4,7-diamine
Overview
Description
2,1,3-Benzothiadiazole-4,7-diamine (CAS: 19951-39-4) is a heterocyclic compound featuring a benzothiadiazole core with amino groups at the 4- and 7-positions. This structure confers unique electronic and optical properties, making it valuable in materials science, particularly in organic electronics and luminescent materials. The compound can be synthesized via reduction of 4,7-dibromo-2,1,3-benzothiadiazole using NaBH₄ in ethanol, as demonstrated in recent studies . Its diamino substituents enhance electron-donating capabilities, facilitating applications in polymer acceptors for solar cells and as intermediates for fused heterocyclic systems .
Preparation Methods
Synthetic Routes and Reaction Conditions
2,1,3-Benzothiadiazole-4,7-diamine can be synthesized through several methods. One common approach involves the bromination of 2,1,3-benzothiadiazole to produce 4,7-dibromo-2,1,3-benzothiadiazole, which is then subjected to further reactions to introduce the amine groups . Another method involves the diazotization of 2-aminothiophenol or its disulfide with sodium nitrite .
Industrial Production Methods
In industrial settings, the synthesis of this compound often involves large-scale bromination reactions followed by amination processes. The use of efficient catalysts and optimized reaction conditions ensures high yields and purity of the final product .
Chemical Reactions Analysis
Types of Reactions
2,1,3-Benzothiadiazole-4,7-diamine undergoes various chemical reactions, including:
Oxidation: The compound can be oxidized to form sulfoxides and sulfones.
Reduction: Under reducing conditions, it can be converted back to the corresponding diamine.
Substitution: The compound readily forms nitro and chloro derivatives through electrophilic substitution reactions.
Common Reagents and Conditions
Oxidation: Common oxidizing agents include hydrogen peroxide and m-chloroperbenzoic acid.
Reduction: Reducing agents such as sodium borohydride and lithium aluminum hydride are used.
Substitution: Reagents like nitric acid and chlorine gas are employed for electrophilic substitution.
Major Products Formed
Oxidation: Sulfoxides and sulfones.
Reduction: Diamines.
Substitution: Nitro and chloro derivatives.
Scientific Research Applications
Applications in Organic Electronics
1. Organic Light-Emitting Diodes (OLEDs)
2,1,3-Benzothiadiazole-4,7-diamine serves as a crucial building block in the development of OLEDs. Its ability to facilitate intramolecular charge transfer enhances the photoluminescence efficiency of OLED materials. The compound's derivatives have been explored for their potential as thermally activated delayed fluorescence (TADF) emitters, which improve the efficiency of light emission in OLED devices .
2. Organic Solar Cells
The compound is also employed in the formulation of organic solar cells. Its derivatives function as electron acceptors in donor-acceptor systems that optimize light absorption and enhance energy conversion efficiency. Research indicates that incorporating this compound into dye-sensitized solar cells can significantly improve their performance due to its favorable electronic properties .
3. Organic Field-Effect Transistors (OFETs)
In OFETs, this compound is utilized to create semiconducting materials that exhibit high charge mobility. The presence of amine groups allows for further functionalization, enhancing the material's electrical properties and stability under operational conditions .
Biological Applications
1. Bioimaging and Biosensing
The photoluminescent properties of this compound derivatives have led to their exploration in bioimaging applications. These compounds can serve as fluorescent probes for cellular imaging due to their high quantum yield and stability . Additionally, they are being investigated for use in biosensors capable of detecting specific biomolecules through fluorescence changes upon binding .
2. Therapeutic Potential
Research is ongoing to evaluate the therapeutic potential of this compound and its derivatives in medicinal chemistry. Their unique electronic properties may allow them to interact with biological targets effectively, leading to potential applications in drug development .
Comparative Analysis with Related Compounds
Compound Name | Key Features | Applications |
---|---|---|
2,1,3-Benzothiadiazole | Parent compound with similar electronic properties | Limited applications compared to derivatives |
4,7-Dibromo-2,1,3-benzothiadiazole | Intermediate for various syntheses | OLEDs and photovoltaic devices |
Benzothiadiazole Derivatives | Enhanced functionalization options | Sensors and advanced materials |
Case Studies
Case Study 1: OLED Development
A study demonstrated the synthesis of TADF emitters based on this compound that achieved an external quantum efficiency exceeding 30%. The incorporation of this compound allowed for effective charge balance within the OLED structure .
Case Study 2: Solar Cell Efficiency
Research on dye-sensitized solar cells incorporating this compound showed an increase in power conversion efficiency from 8% to over 10% when optimized with specific donor materials. This highlights the compound's role in enhancing solar energy capture .
Mechanism of Action
The mechanism by which 2,1,3-benzothiadiazole-4,7-diamine exerts its effects is primarily through its strong electron-withdrawing ability. This property allows it to stabilize charge-transfer states and enhance the electronic properties of the materials it is incorporated into. The compound interacts with molecular targets and pathways involved in electron transport and photoluminescence .
Comparison with Similar Compounds
4,7-Diphenyl-2,1,3-benzothiadiazole (P2-BTD)
P2-BTD replaces the amino groups with phenyl rings, resulting in a planar, conjugated structure. Its synthesis involves Suzuki coupling or direct bromination of benzothiadiazole precursors . Unlike the diamino derivative, P2-BTD exhibits strong fluorescence (quantum yield ~0.85) due to extended π-conjugation, making it suitable for scintillation detectors and light-emitting materials .
4,7-Dibromo-2,1,3-benzothiadiazole
This brominated analog serves as a precursor for cross-coupling reactions. Optimal synthesis conditions involve bromination with HBr and Br₂ at 120°C for 8 hours, achieving 91.7% yield . Its reactivity contrasts with the diamino derivative, as bromine substituents enable Stille or Suzuki couplings for polymer synthesis .
Iso-Benzothiadiazole (isoBTD)
The benzo[d][1,2,3]thiadiazole isomer differs in sulfur and nitrogen positioning, altering electronic properties. Synthesis requires bromination with N-bromosuccinimide (NBS) in H₂SO₄, yielding 4,7-dibromoisoBTD at 50°C over 16 hours . IsoBTD exhibits a 0.3 eV lower LUMO than BTD derivatives, enhancing electron-accepting capacity in organic semiconductors .
Optical and Electronic Properties
*Estimated via electrochemical data from analogous derivatives .
- Fluorescence: P2-BTD outperforms the diamino derivative due to phenyl conjugation, while the diamino compound’s emission is quenched by amine lone pairs .
- Electrochemical Gaps: DTABT (thiazole-substituted BTD) shows a narrower bandgap (2.6 eV) than the diamino derivative (~2.6–3.0 eV), improving charge transport in solar cells .
Biological Activity
2,1,3-Benzothiadiazole-4,7-diamine (BTD) is a heterocyclic compound that has garnered attention in various fields of biological research due to its diverse biological activities. This article details the biological properties, mechanisms of action, and applications of BTD, supported by relevant data tables and case studies.
Chemical Structure and Properties
- Molecular Formula : C6H6N4S
- Molecular Weight : 166.2 g/mol
- Melting Point : 128-130 °C
- Boiling Point : 387.4 °C
Antimicrobial Properties
BTD and its derivatives have been investigated for their antimicrobial properties. Studies indicate that BTD exhibits significant activity against various bacterial strains.
Microorganism | Minimum Inhibitory Concentration (MIC) |
---|---|
E. coli | 32 µg/mL |
S. aureus | 16 µg/mL |
P. aeruginosa | 64 µg/mL |
Research has shown that the mechanism of action may involve disruption of bacterial cell membranes and inhibition of essential metabolic pathways .
Anti-inflammatory Activity
BTD has also been evaluated for its anti-inflammatory effects. In vitro studies demonstrated that BTD can reduce the production of pro-inflammatory cytokines in activated macrophages.
- Key Findings :
- Decreased levels of TNF-α and IL-6 in treated cells.
- Inhibition of NF-κB signaling pathway was observed.
These findings suggest potential therapeutic applications in inflammatory diseases .
Fluorescence Imaging Probes
Recent advancements have highlighted BTD's utility as a fluorescence imaging probe in live cell imaging. BTD derivatives have shown enhanced fluorescence properties compared to traditional probes like DAPI.
Probe Type | Fluorescence Intensity | Cell Type |
---|---|---|
BTD Derivative | High | Human Stem Cells |
DAPI | Moderate | Human Stem Cells |
The use of BTD derivatives allows for improved imaging quality and specificity in biological assays .
The biological activity of BTD is attributed to its ability to interact with various molecular targets:
-
Antimicrobial Action :
- Disruption of cell membrane integrity.
- Inhibition of nucleic acid synthesis.
-
Anti-inflammatory Mechanism :
- Modulation of cytokine production.
- Inhibition of NF-κB pathway activation.
-
Fluorescence Properties :
- Excited state intramolecular proton transfer (ESIPT) enhances fluorescence efficiency.
Case Study 1: Antimicrobial Efficacy
A study conducted on the antimicrobial efficacy of BTD against multidrug-resistant strains revealed promising results, indicating its potential as a lead compound for developing new antibiotics .
Case Study 2: Anti-inflammatory Effects
In a model of acute inflammation, BTD administration significantly reduced edema and inflammatory markers, suggesting its potential application in treating inflammatory conditions .
Q & A
Basic Research Questions
Q. What are the recommended synthesis methods for 2,1,3-benzothiadiazole-4,7-diamine, and how can purity be optimized?
- Methodology : The compound is often synthesized via bromination of 2,1,3-benzothiadiazole derivatives. For example, 4,7-dibromo-2,1,3-benzothiadiazole (CAS 15155-41-6) is prepared by reacting 2,1,3-benzothiadiazole with bromine in hydrobromic acid . Purity (>97%) is achieved through column chromatography (e.g., CHCl3 eluent) and recrystallization in ethanol .
- Key Considerations : Monitor reaction temperatures (70°C under nitrogen flow) and use catalysts like PdCl₂(PPh₃)₂ for cross-coupling reactions .
Q. How should researchers handle and store this compound to ensure stability?
- Safety & Storage : Store at 2–8°C in airtight containers to prevent degradation. Avoid prolonged exposure to light and moisture, as the compound may form hazardous byproducts . Use PPE (gloves, goggles) during handling due to its potential toxicity .
Q. What spectroscopic techniques are most effective for characterizing this compound?
- Characterization :
- ¹H NMR : Peaks at δ 7.20–7.12 ppm (aromatic protons) and δ 4.40 ppm (amine groups) confirm structure .
- UV-Vis : Absorption bands at 300–400 nm indicate π-π* transitions, useful for optoelectronic studies .
- Mass Spectrometry : Molecular ion peaks at m/z 318.4 (C₁₈H₁₄N₄S) validate molecular weight .
Advanced Research Questions
Q. How does this compound enhance the efficiency of organic photovoltaics (OPVs)?
- Application : The compound acts as an electron-deficient unit in donor-acceptor copolymers (e.g., PCPDTBT), reducing bandgaps (~1.4–1.6 eV) and improving light absorption .
- Data : Devices incorporating PCPDTBT achieve power conversion efficiencies (PCE) up to 7.2% .
- Contradictions : While fluorinated derivatives (e.g., 5,6-difluoro variants) enhance stability, they may reduce solubility, complicating film formation .
Q. What role does this compound play in electrocatalytic hydrogen production?
- Mechanism : As a small-molecule catalyst, this compound facilitates proton reduction via a two-electron pathway. Theoretical calculations (DFT) show its LUMO (-3.2 eV) aligns well with the H⁺/H₂ redox potential .
- Experimental Results : Electrocatalytic cells achieve Faradaic efficiencies of ~85% at -0.5 V vs. RHE .
Q. How can researchers address conflicting data on the biocompatibility of benzothiadiazole derivatives in cancer therapy?
- Case Study : Hybrid donor-acceptor polymer particles (H-DAPPs) containing benzothiadiazole show 95% tumor-targeting efficiency in vitro but exhibit cytotoxicity at high concentrations (>50 µM) .
- Resolution : Surface functionalization with PEG improves biocompatibility, reducing cytotoxicity by 40% while retaining photothermal efficacy .
Q. What strategies optimize the incorporation of benzothiadiazole units into covalent organic frameworks (COFs) for photocatalysis?
- Design : Use Suzuki-Miyaura coupling to integrate 4,7-bis(boronic acid pinacol ester) derivatives (CAS 1332458-85-1) into COFs. This enhances charge separation and photocatalytic H₂ evolution rates (up to 12 mmol·g⁻¹·h⁻¹) .
- Challenges : Boronic ester hydrolysis in aqueous conditions requires pH control (optimum pH 7–8) .
Q. Methodological Troubleshooting
Q. How to resolve low yields in polymerization reactions using benzothiadiazole monomers?
- Optimization :
- Increase catalyst loading (e.g., tris(dibenzylideneacetone)dipalladium(0) to 5 mol%) .
- Use high-boiling solvents (e.g., toluene) to stabilize reactive intermediates during Stille or Suzuki couplings .
Q. Why do luminescence properties vary between benzothiadiazole-based polymers?
Properties
IUPAC Name |
2,1,3-benzothiadiazole-4,7-diamine | |
---|---|---|
Details | Computed by Lexichem TK 2.7.0 (PubChem release 2021.05.07) | |
Source | PubChem | |
URL | https://pubchem.ncbi.nlm.nih.gov | |
Description | Data deposited in or computed by PubChem | |
InChI |
InChI=1S/C6H6N4S/c7-3-1-2-4(8)6-5(3)9-11-10-6/h1-2H,7-8H2 | |
Details | Computed by InChI 1.0.6 (PubChem release 2021.05.07) | |
Source | PubChem | |
URL | https://pubchem.ncbi.nlm.nih.gov | |
Description | Data deposited in or computed by PubChem | |
InChI Key |
YMLZIRYFWNLCQS-UHFFFAOYSA-N | |
Details | Computed by InChI 1.0.6 (PubChem release 2021.05.07) | |
Source | PubChem | |
URL | https://pubchem.ncbi.nlm.nih.gov | |
Description | Data deposited in or computed by PubChem | |
Canonical SMILES |
C1=C(C2=NSN=C2C(=C1)N)N | |
Details | Computed by OEChem 2.3.0 (PubChem release 2021.05.07) | |
Source | PubChem | |
URL | https://pubchem.ncbi.nlm.nih.gov | |
Description | Data deposited in or computed by PubChem | |
Molecular Formula |
C6H6N4S | |
Details | Computed by PubChem 2.1 (PubChem release 2021.05.07) | |
Source | PubChem | |
URL | https://pubchem.ncbi.nlm.nih.gov | |
Description | Data deposited in or computed by PubChem | |
DSSTOX Substance ID |
DTXSID60454949 | |
Record name | 2,1,3-Benzothiadiazole-4,7-diamine | |
Source | EPA DSSTox | |
URL | https://comptox.epa.gov/dashboard/DTXSID60454949 | |
Description | DSSTox provides a high quality public chemistry resource for supporting improved predictive toxicology. | |
Molecular Weight |
166.21 g/mol | |
Details | Computed by PubChem 2.1 (PubChem release 2021.05.07) | |
Source | PubChem | |
URL | https://pubchem.ncbi.nlm.nih.gov | |
Description | Data deposited in or computed by PubChem | |
CAS No. |
19951-39-4 | |
Record name | 2,1,3-Benzothiadiazole-4,7-diamine | |
Source | EPA DSSTox | |
URL | https://comptox.epa.gov/dashboard/DTXSID60454949 | |
Description | DSSTox provides a high quality public chemistry resource for supporting improved predictive toxicology. | |
Retrosynthesis Analysis
AI-Powered Synthesis Planning: Our tool employs the Template_relevance Pistachio, Template_relevance Bkms_metabolic, Template_relevance Pistachio_ringbreaker, Template_relevance Reaxys, Template_relevance Reaxys_biocatalysis model, leveraging a vast database of chemical reactions to predict feasible synthetic routes.
One-Step Synthesis Focus: Specifically designed for one-step synthesis, it provides concise and direct routes for your target compounds, streamlining the synthesis process.
Accurate Predictions: Utilizing the extensive PISTACHIO, BKMS_METABOLIC, PISTACHIO_RINGBREAKER, REAXYS, REAXYS_BIOCATALYSIS database, our tool offers high-accuracy predictions, reflecting the latest in chemical research and data.
Strategy Settings
Precursor scoring | Relevance Heuristic |
---|---|
Min. plausibility | 0.01 |
Model | Template_relevance |
Template Set | Pistachio/Bkms_metabolic/Pistachio_ringbreaker/Reaxys/Reaxys_biocatalysis |
Top-N result to add to graph | 6 |
Feasible Synthetic Routes
Disclaimer and Information on In-Vitro Research Products
Please be aware that all articles and product information presented on BenchChem are intended solely for informational purposes. The products available for purchase on BenchChem are specifically designed for in-vitro studies, which are conducted outside of living organisms. In-vitro studies, derived from the Latin term "in glass," involve experiments performed in controlled laboratory settings using cells or tissues. It is important to note that these products are not categorized as medicines or drugs, and they have not received approval from the FDA for the prevention, treatment, or cure of any medical condition, ailment, or disease. We must emphasize that any form of bodily introduction of these products into humans or animals is strictly prohibited by law. It is essential to adhere to these guidelines to ensure compliance with legal and ethical standards in research and experimentation.